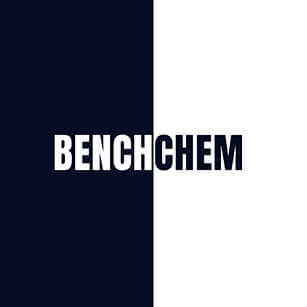
Methyl D-Glucuronate
- Click on QUICK INQUIRY to receive a quote from our team of experts.
- With the quality product at a COMPETITIVE price, you can focus more on your research.
Overview
Description
Methyl D-Glucuronate is a derivative of D-glucuronic acid, a glucose derivative. It is known for its various applications in the fields of medicine, chemistry, and industry due to its unique chemical properties. The compound has a molecular formula of C7H12O7 and a molecular weight of 208.17 .
Mechanism of Action
Target of Action
Methyl D-Glucuronate primarily targets enzymes involved in the metabolism of glucose derivatives . These enzymes include glucuronoyl esterases (GEs), which belong to the carbohydrate esterase family 15 (CE15) . GEs play a central role in the reduction of recalcitrance in plant cell walls by cleaving ester linkages between glucuronoxylan and lignin in lignocellulose .
Mode of Action
This compound interacts with its targets, primarily the GEs, to facilitate the cleavage of ester-linked lignin-carbohydrate complexes . This interaction is crucial for the integrity of plant cell walls and potentially interacts with lignin itself .
Biochemical Pathways
This compound affects the pentose and glucuronate interconversion pathways . It acts as a key molecule to bind toxic substances via these pathways . In addition, it is involved in the production of D-glucuronic acid through various methods, including single enzyme catalysis, multi-enzyme cascade, whole cell catalysis, and co-culture .
Pharmacokinetics
The production of d-glucuronic acid, a glucose derivative, by biocatalysis has been identified as a promising alternative method due to its high efficiency and environmental friendliness .
Result of Action
The molecular and cellular effects of this compound’s action are primarily seen in its role in facilitating the cleavage of ester-linked lignin-carbohydrate complexes . This results in the reduction of recalcitrance in plant cell walls, which is crucial for the integrity of these structures .
Biochemical Analysis
Biochemical Properties
Methyl D-Glucuronate can be generated through the oxidation of the primary alcohol hydroxyl group of glucose into a carboxyl group . It widely exists in plants and animals in the form of tissue complexes . For example, chondroitin sulfate in human connective tissues and Callaya resin glue in plants are rich in this compound .
Cellular Effects
This compound has excellent moisturizing properties and biocompatibility . It is found mainly in connective tissue, skin, cartilage and eyeball of the human body . It can absorb and lock in moisture, and each gram of this compound can absorb at least 1000 ml of water, keeping the skin moist .
Molecular Mechanism
This compound has anti-inflammatory and antibacterial effects . It has important applications in the medical treatment of liver diseases . This compound and its derivatives can combine with endogenous and exogenous toxic substances such as phenol and hydroxyl and amino and alcohol groups in the liver to produce uronic acid substances , thus increasing the water solubility and promoting their excretion through renal urine or sweat .
Temporal Effects in Laboratory Settings
The traditional production methods of this compound mainly include natural extraction and chemical synthesis, which can no longer meet the growing market demand . The production of this compound by biocatalysis has become a promising alternative method because of its high efficiency and environmental friendliness .
Metabolic Pathways
This compound can be generated through the oxidation of the primary alcohol hydroxyl group of glucose into a carboxyl group . This indicates that it is involved in the metabolic pathway of glucose oxidation.
Preparation Methods
Synthetic Routes and Reaction Conditions
Methyl D-Glucuronate can be synthesized through several methods. One common approach involves the oxidation of D-glucose derivatives. For instance, the oxidation of 1-O-methyl-D-glucose with potassium permanganate can yield this compound . Another method involves the use of glucosyluronate bromide in the presence of silver oxide to achieve glucuronidation .
Industrial Production Methods
Industrial production of D-glucuronic acid, from which this compound is derived, typically involves chemical oxidation, polysaccharide hydrolysis, and biological catalysis. Chemical oxidation is the most commonly used method, involving the oxidation of starch by concentrated nitric acid and subsequent hydrolysis under high pressure .
Chemical Reactions Analysis
Types of Reactions
Methyl D-Glucuronate undergoes various chemical reactions, including oxidation, reduction, and substitution. These reactions are essential for its applications in different fields.
Common Reagents and Conditions
Oxidation: Potassium permanganate is commonly used as an oxidizing agent.
Reduction: Specific reducing agents can be employed depending on the desired product.
Substitution: Reagents such as trichloroacetonitrile and 1,8-diazabicyclo[5,4,0]-undec-7-ene (DBU) are used in substitution reactions.
Major Products Formed
The major products formed from these reactions include various glucuronides and their derivatives, which have significant biological and chemical applications .
Scientific Research Applications
Methyl D-Glucuronate and its derivatives have been extensively studied for their antitubercular, antimicrobial, and hemolytic activities. These compounds have shown high antitubercular activity comparable to isoniazid . Additionally, D-glucuronic acid, from which this compound is derived, has applications in medicine, cosmetics, and food due to its anti-oxidation properties and its role in treating liver diseases and hyperlipidemia .
Comparison with Similar Compounds
Methyl D-Glucuronate can be compared with other similar compounds such as glucuronic acid, galacturonic acid, and mannuronic acid. These compounds share similar chemical structures but differ in their specific applications and properties. For example, glucuronic acid is widely used in the synthesis of glycosides and oligosaccharides, while galacturonic acid and mannuronic acid have unique applications in the synthesis of bioactive molecules and complex oligosaccharides .
Conclusion
This compound is a versatile compound with significant applications in various fields. Its unique chemical properties and ability to undergo various reactions make it valuable for scientific research and industrial applications. Understanding its preparation methods, chemical reactions, and mechanism of action can further enhance its utilization in medicine, chemistry, and industry.
Properties
CAS No. |
52613-19-1 |
---|---|
Molecular Formula |
C7H12O7 |
Molecular Weight |
208.17 g/mol |
IUPAC Name |
methyl (2S,3S,5R)-3,4,5,6-tetrahydroxyoxane-2-carboxylate |
InChI |
InChI=1S/C7H12O7/c1-13-7(12)5-3(9)2(8)4(10)6(11)14-5/h2-6,8-11H,1H3/t2?,3-,4+,5-,6?/m0/s1 |
InChI Key |
DICCNWCUKCYGNF-CQGHMCOMSA-N |
SMILES |
COC(=O)C1C(C(C(C(O1)O)O)O)O |
Isomeric SMILES |
COC(=O)[C@@H]1[C@H](C([C@H](C(O1)O)O)O)O |
Canonical SMILES |
COC(=O)C1C(C(C(C(O1)O)O)O)O |
Synonyms |
D-Glucuronic Acid Methyl Ester; D-Glucuronic Acid Methyl Ester; |
Origin of Product |
United States |
Q1: How does Methyl D-Glucuronate contribute to the development of Carbonic Anhydrase inhibitors?
A1: this compound serves as a sugar moiety in the synthesis of glycoconjugate benzene sulfonamides, which have shown potential as Carbonic Anhydrase (CA) inhibitors []. These inhibitors are synthesized by linking the CA pharmacophore (ArSO2NH2) to this compound through a triazole linker. The resulting glycoconjugates demonstrate varying degrees of potency and selectivity against different CA isozymes, suggesting that modifications to the sugar moiety can fine-tune their inhibitory properties.
Q2: Can you provide a specific example of a potent CA inhibitor derived from this compound and its target?
A2: Yes, compound 6, a this compound triazole, exhibited strong inhibitory activity against human Carbonic Anhydrase IX (hCA IX) with a Ki of 9.9 nM []. This selectivity towards hCA IX, a tumor-associated isozyme, suggests potential applications in targeted cancer therapies.
Q3: Besides CA inhibition, are there other enzymatic interactions involving this compound?
A3: Yes, this compound, specifically its uridine diphosphate derivative (UDP-Methyl-D-Glucuronate), acts as a competitive inhibitor for UDP-apiose/UDP-xylose synthase []. This enzyme, found in parsley cell cultures, utilizes UDP-D-Glucuronic acid (UDP-GlcUA) as its natural substrate.
Q4: What is the significance of UDP-Methyl-D-Glucuronate's inhibitory action on UDP-apiose/UDP-xylose synthase?
A4: This inhibition provides insights into the enzyme's substrate specificity and reaction mechanism []. The fact that UDP-Methyl-D-Glucuronate, structurally similar to UDP-GlcUA, can bind to the enzyme but not be converted suggests that the methyl group at a specific position hinders the enzymatic reaction.
Q5: What analytical techniques have been used to study this compound and its derivatives?
A5: Various spectroscopic methods have been employed. For instance, difference spectroscopy was used to observe the transient formation of a 4-keto derivative during the reaction of UDP-apiose/UDP-xylose synthase with UDP-GlcUA, UDP-Methyl-D-Glucuronate, and UDP-Glc []. Additionally, the formation of stable 4-keto derivatives upon reaction with o-phenylenediamine was monitored through UV-Vis spectrophotometry []. While these studies focused on the UDP derivative, they highlight the application of spectroscopic methods in studying the reactivity and interactions of this compound derivatives.
Q6: How does the structure of this compound impact its biological activity?
A6: Although specific structure-activity relationship (SAR) studies for this compound itself weren't detailed in the provided papers, research on glycoconjugate CA inhibitors highlights the importance of the sugar moiety's structure []. Modifications to the ring size, stereochemistry, and chain length of the sugar tail, which in this case includes this compound, significantly influenced the potency and selectivity of the resulting inhibitors. This suggests that future research focusing on systematically modifying the this compound structure could further optimize its interaction with target enzymes and unlock its full therapeutic potential.
Disclaimer and Information on In-Vitro Research Products
Please be aware that all articles and product information presented on BenchChem are intended solely for informational purposes. The products available for purchase on BenchChem are specifically designed for in-vitro studies, which are conducted outside of living organisms. In-vitro studies, derived from the Latin term "in glass," involve experiments performed in controlled laboratory settings using cells or tissues. It is important to note that these products are not categorized as medicines or drugs, and they have not received approval from the FDA for the prevention, treatment, or cure of any medical condition, ailment, or disease. We must emphasize that any form of bodily introduction of these products into humans or animals is strictly prohibited by law. It is essential to adhere to these guidelines to ensure compliance with legal and ethical standards in research and experimentation.