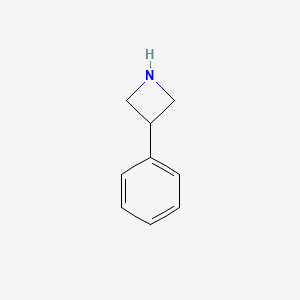
3-Phenylazetidine
Overview
Description
3-Phenylazetidine is a useful research compound. Its molecular formula is C9H11N and its molecular weight is 133.194. The purity is usually 95%.
BenchChem offers high-quality this compound suitable for many research applications. Different packaging options are available to accommodate customers' requirements. Please inquire for more information about this compound including the price, delivery time, and more detailed information at info@benchchem.com.
Mechanism of Action
Target of Action
It is known that azetidines, the class of compounds to which 3-phenylazetidine belongs, are often used in medicinal chemistry due to their ability to efficiently tune the pharmacological properties of molecules .
Mode of Action
Azetidines are known to be synthesized through various methods, including the aza paternò–büchi reaction . This reaction involves a [2 + 2] photocycloaddition between an imine and an alkene component, resulting in the formation of functionalized azetidines .
Biochemical Pathways
Azetidines are known to be used as building blocks for polyamines through anionic and cationic ring-opening polymerization . This suggests that this compound may play a role in the synthesis of polyamines, which are involved in various biological processes.
Result of Action
Azetidines are known to be used in the synthesis of complex natural products . This suggests that this compound may have a role in the synthesis of these products, potentially influencing their molecular and cellular effects.
Action Environment
Environmental factors can influence the action, efficacy, and stability of many compounds . .
Properties
IUPAC Name |
3-phenylazetidine | |
---|---|---|
Source | PubChem | |
URL | https://pubchem.ncbi.nlm.nih.gov | |
Description | Data deposited in or computed by PubChem | |
InChI |
InChI=1S/C9H11N/c1-2-4-8(5-3-1)9-6-10-7-9/h1-5,9-10H,6-7H2 | |
Source | PubChem | |
URL | https://pubchem.ncbi.nlm.nih.gov | |
Description | Data deposited in or computed by PubChem | |
InChI Key |
XOZGEXKQMVAILQ-UHFFFAOYSA-N | |
Source | PubChem | |
URL | https://pubchem.ncbi.nlm.nih.gov | |
Description | Data deposited in or computed by PubChem | |
Canonical SMILES |
C1C(CN1)C2=CC=CC=C2 | |
Source | PubChem | |
URL | https://pubchem.ncbi.nlm.nih.gov | |
Description | Data deposited in or computed by PubChem | |
Molecular Formula |
C9H11N | |
Source | PubChem | |
URL | https://pubchem.ncbi.nlm.nih.gov | |
Description | Data deposited in or computed by PubChem | |
DSSTOX Substance ID |
DTXSID30651786 | |
Record name | 3-Phenylazetidine | |
Source | EPA DSSTox | |
URL | https://comptox.epa.gov/dashboard/DTXSID30651786 | |
Description | DSSTox provides a high quality public chemistry resource for supporting improved predictive toxicology. | |
Molecular Weight |
133.19 g/mol | |
Source | PubChem | |
URL | https://pubchem.ncbi.nlm.nih.gov | |
Description | Data deposited in or computed by PubChem | |
CAS No. |
4363-13-7 | |
Record name | 3-Phenylazetidine | |
Source | EPA DSSTox | |
URL | https://comptox.epa.gov/dashboard/DTXSID30651786 | |
Description | DSSTox provides a high quality public chemistry resource for supporting improved predictive toxicology. | |
Synthesis routes and methods I
Procedure details
Synthesis routes and methods II
Procedure details
Q1: What are some synthetic routes to access 3-phenylazetidine derivatives?
A1: Several methods have been explored for the synthesis of this compound derivatives:
- Strained ring opening: 3-Phenyl-1-azabicyclo[1.1.0]butane reacts with chlorodithioformates to yield 3-chloro-3-phenylazetidine-1-carbodithioates. Further derivatization allows access to azetidinethiocarboxylate derivatives. []
- Cycloaddition reactions: Microwave-assisted reactions of 1-arenesulfonylaziridines with dimethylsulfonium carboethoxymethylide, using alumina as a solid support, lead to the regioselective synthesis of trans-1-arenesulfonyl-2-ethoxycarbonyl-3-phenylazetidines. []
- Photocyclization: Irradiation of bis(phenylglyoxyloyl)alkylamines induces cyclization involving a 1,5-benzoyl shift, forming 1-alkyl-3-benzoyloxy-3 phenylazetidine-2,4-diones. []
Q2: How does the strained ring system of 1-azabicyclo[1.1.0]butanes contribute to the synthesis of azetidines?
A: The high ring strain in 1-azabicyclo[1.1.0]butanes makes them highly reactive towards ring-opening reactions. This reactivity is exploited in their reaction with trimethylsilyl azide, where the strained N-C(3) bond is cleaved, leading to the formation of 3-azido-3-ethylazetidine derivatives after subsequent reactions with various electrophiles. []
Q3: What is the significance of synthesizing cis- and trans-3-phenylazetidine-2-carboxylic acids?
A: These compounds are conformationally restricted analogues of phenylalanine, a naturally occurring amino acid. Studying their synthesis and properties can provide valuable insights into the structure-activity relationships of phenylalanine analogues and their potential use as tools in medicinal chemistry. [, ]
Q4: Can you describe a specific example of how the this compound structure has been modified and the observed outcome?
A: Researchers investigated the synthesis of 3-hydroxymethyl-3-phenylazetidine derivatives and attempted to convert them into more complex bicyclic systems like 3-phenyl-1-azabicyclo[1.1.1]pentane or 3-phenyl-1-azabicyclo[2.1.1]hexane. This research highlights the potential of modifying the this compound scaffold to generate new and potentially bioactive compounds. []
Q5: What crystallographic information is available for this compound derivatives?
A: The crystal structure of 1-N-Methyl-2[1′-N-(p-methoxyphenyl)-3′-phenylazetidine-2′-one]-3-(p-methoxybenzoyl)spiro[4.4″]-oxindole-pyrrolidine has been determined. This complex molecule, containing a this compound moiety, reveals structural features such as the conformation of the pyrrolidine ring and stabilizing intramolecular and intermolecular interactions. []
Q6: Has the concept of azidonium intermediates, potentially relevant to azetidine synthesis, been explored?
A: Yes, research simulating azidonium intermediates using N-aminoheterocycles, including azetidine derivatives, has been conducted. While not directly leading to azetidine synthesis in the reported cases, this work provides insights into the reactivity and stability of potential intermediates relevant to azetidine chemistry. []
Disclaimer and Information on In-Vitro Research Products
Please be aware that all articles and product information presented on BenchChem are intended solely for informational purposes. The products available for purchase on BenchChem are specifically designed for in-vitro studies, which are conducted outside of living organisms. In-vitro studies, derived from the Latin term "in glass," involve experiments performed in controlled laboratory settings using cells or tissues. It is important to note that these products are not categorized as medicines or drugs, and they have not received approval from the FDA for the prevention, treatment, or cure of any medical condition, ailment, or disease. We must emphasize that any form of bodily introduction of these products into humans or animals is strictly prohibited by law. It is essential to adhere to these guidelines to ensure compliance with legal and ethical standards in research and experimentation.