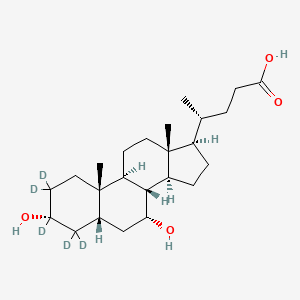
Chenodeoxycholic Acid-d5 (Major)
Overview
Description
Chenodeoxycholic Acid-d5 (Major) is a deuterated form of chenodeoxycholic acid, a primary bile acid synthesized in the liver from cholesterol. It is used as a stable isotope-labeled compound in various scientific research applications. The deuterium atoms replace hydrogen atoms in the molecule, making it useful for tracing and studying metabolic pathways.
Preparation Methods
Synthetic Routes and Reaction Conditions
Chenodeoxycholic Acid-d5 (Major) is synthesized through a series of chemical reactions starting from 3α,7α-dihydroxy-5β-cholanic acid. The process involves forming an intermediate compound, which is then subjected to further chemical reactions to produce the final product . The conditions for each step are carefully controlled to ensure high yield and purity.
Industrial Production Methods
The industrial production of Chenodeoxycholic Acid-d5 (Major) involves large-scale synthesis using similar methods as in laboratory settings but optimized for efficiency and cost-effectiveness. The process includes extraction, purification, and crystallization steps to obtain the final product in its pure form .
Chemical Reactions Analysis
Types of Reactions
Chenodeoxycholic Acid-d5 (Major) undergoes various chemical reactions, including:
Oxidation: Conversion of hydroxyl groups to ketones or carboxylic acids.
Reduction: Reduction of ketones to hydroxyl groups.
Substitution: Replacement of hydroxyl groups with other functional groups.
Common Reagents and Conditions
Common reagents used in these reactions include oxidizing agents like potassium permanganate, reducing agents like sodium borohydride, and substitution reagents like thionyl chloride. The reactions are typically carried out under controlled temperatures and pH conditions to ensure specificity and yield.
Major Products
The major products formed from these reactions include various derivatives of Chenodeoxycholic Acid-d5 (Major), such as ketones, alcohols, and esters, which are used in further research and applications.
Scientific Research Applications
Chenodeoxycholic Acid-d5 (Major) has a wide range of scientific research applications, including:
Chemistry: Used as a tracer in metabolic studies to understand the pathways and transformations of bile acids.
Biology: Helps in studying the role of bile acids in cellular processes and their interactions with receptors.
Medicine: Used in the development of drugs targeting bile acid pathways and in the treatment of liver and gallbladder diseases.
Industry: Applied in the synthesis of other bile acid derivatives and in the production of pharmaceuticals
Mechanism of Action
Chenodeoxycholic Acid-d5 (Major) exerts its effects by interacting with specific molecular targets and pathways. It binds to the farnesoid X receptor (FXR) and G protein-coupled bile acid receptor 1 (GPBAR1), modulating the expression of genes involved in bile acid synthesis, cholesterol metabolism, and glucose homeostasis . This interaction helps in regulating bile acid levels and maintaining metabolic balance.
Comparison with Similar Compounds
Similar Compounds
Chenodeoxycholic Acid: The non-deuterated form, used in similar applications but without the benefits of stable isotope labeling.
Ursodeoxycholic Acid: Another bile acid used in the treatment of gallstones and liver diseases.
Lithocholic Acid: A secondary bile acid with different metabolic and physiological effects.
Uniqueness
Chenodeoxycholic Acid-d5 (Major) is unique due to its stable isotope labeling, which allows for precise tracing and quantification in metabolic studies. This makes it a valuable tool in research compared to its non-deuterated counterparts .
Biological Activity
Chenodeoxycholic acid-d5 (CDCA-d5) is a deuterated form of chenodeoxycholic acid (CDCA), a bile acid that plays significant roles in lipid metabolism, cholesterol regulation, and liver function. This article delves into the biological activities of CDCA-d5, highlighting its mechanisms of action, therapeutic applications, and relevant research findings.
Overview of Chenodeoxycholic Acid
Chenodeoxycholic Acid (CDCA) is a primary bile acid synthesized in the liver from cholesterol. It is crucial for the emulsification and absorption of dietary fats and fat-soluble vitamins in the intestine. CDCA acts as a signaling molecule, activating various nuclear receptors such as the farnesoid X receptor (FXR), which regulates bile acid synthesis and glucose metabolism.
-
Cholesterol Regulation :
- CDCA is a potent inhibitor of cholesterol 7α-hydroxylase, the rate-limiting enzyme in bile acid synthesis. Studies show that treatment with CDCA significantly reduces serum levels of 7α-hydroxy-4-cholesten-3-one, indicating decreased cholesterol synthesis .
- CDCA also suppresses hepatic 3-hydroxy-3-methyl glutaryl CoA (HMG-CoA) reductase activity, further inhibiting cholesterol production .
-
Inflammation and Fibrosis :
- Research indicates that CDCA can activate the NLRP3 inflammasome in macrophages, leading to the maturation and release of interleukin-1β (IL-1β), a pro-inflammatory cytokine. This activation suggests a role for CDCA in cholestatic liver injury .
- Inhibition of the inflammasome may mitigate liver fibrosis in cholestasis models, highlighting CDCA's dual role in promoting inflammation while also being involved in liver injury responses .
- Bile Acid Signaling :
Therapeutic Applications
CDCA has several clinical applications:
- Gallstone Dissolution : CDCA is used to dissolve cholesterol gallstones in patients who are not candidates for surgery. It facilitates the breakdown of cholesterol stones by altering bile composition .
- Cerebrotendineous Xanthomatosis : This rare genetic disorder characterized by abnormal bile acid synthesis can be treated with CDCA to manage symptoms effectively .
- Potential Use in Hepatitis C : A combination therapy involving CDCA and bezafibrate has been investigated for its efficacy against hepatitis C infection, showing promising results in preliminary studies .
Table 1: Summary of Key Research Findings on CDCA-d5
Case Study: Cholestatic Liver Injury
In a study involving bile duct ligation (BDL) mouse models, CDCA was shown to exacerbate liver injury through NLRP3 inflammasome activation. The inhibition of this pathway resulted in reduced fibrosis markers, suggesting that targeting this mechanism may provide therapeutic benefits for patients with cholestatic liver diseases .
Q & A
Basic Research Questions
Q. What methodological advantages does Chenodeoxycholic Acid-d5 (CDCA-d5) offer in bile acid metabolism studies?
CDCA-d5 is a deuterium-labeled analog of chenodeoxycholic acid (CDCA), enabling precise tracking of bile acid kinetics using techniques like mass spectrometry (MS) or nuclear magnetic resonance (NMR). Its isotopic labeling allows researchers to distinguish endogenous CDCA from administered doses in in vivo or in vitro systems, minimizing background noise in metabolic flux analyses. For example, CDCA-d5 can be used to quantify enterohepatic recirculation efficiency or assess compartment-specific metabolism (e.g., hepatic vs. intestinal) .
Q. How can CDCA-d5 improve the accuracy of bile acid quantification in complex biological matrices?
CDCA-d5 serves as an internal standard in liquid chromatography-tandem MS (LC-MS/MS) workflows. By spiking known concentrations into samples, researchers correct for matrix effects and ionization variability. This is critical in studies where endogenous bile acids (e.g., CDCA, cholic acid) share similar fragmentation patterns, as CDCA-d5’s distinct mass shift (+5 Da) ensures unambiguous identification .
Q. What are the key considerations for maintaining CDCA-d5 stability in experimental setups?
CDCA-d5 should be stored at +4°C to prevent degradation . In cell culture or animal studies, solubility challenges (due to its hydrophobic nature) may require formulation with cyclodextrins or bile acid transporters. Pre-experiment validation via HPLC or MS is recommended to confirm isotopic purity (>95%) and rule out deuterium exchange under physiological conditions .
Advanced Research Questions
Q. How can researchers address conflicting data on CDCA-d5’s receptor activation profiles across different models?
CDCA activates nuclear receptors like FXR and membrane receptors like TGR5, but deuterium substitution in CDCA-d5 may subtly alter binding kinetics. To resolve discrepancies, conduct parallel dose-response assays comparing CDCA and CDCA-d5 in recombinant receptor systems (e.g., luciferase reporter assays) and primary hepatocytes. Cross-validate findings with isotopic tracing to confirm target engagement .
Q. What experimental designs mitigate limitations in clinical studies involving CDCA-d5, such as small sample sizes or retrospective bias?
For preclinical-to-clinical translation:
- Use CDCA-d5 in longitudinal, placebo-controlled trials with stratified cohorts (e.g., NAFLD patients).
- Combine isotopic tracing with multi-omics (metabolomics, proteomics) to capture systemic effects beyond bile acid metabolism.
- Leverage retrospective clinical data (e.g., from CTX studies ) to inform power calculations and endpoint selection .
Q. How can CDCA-d5 elucidate the gut-liver axis in metabolic disorders?
Incorporate CDCA-d5 into gnotobiotic mouse models or human gut-microbiome co-cultures to track microbial modification (e.g., deconjugation, 7α-dehydroxylation). Pair with 16S rRNA sequencing to correlate isotopic enrichment with microbial taxa. For example, CDCA-d5 can identify bacterial strains responsible for converting primary to secondary bile acids, linking dysbiosis to hepatic inflammation .
Q. What analytical challenges arise when quantifying CDCA-d5 in the presence of isomeric bile acids, and how can they be resolved?
Isomeric interference (e.g., from ursodeoxycholic acid or allobil esters) can skew CDCA-d5 measurements. Solutions include:
- Using ultrahigh-resolution MS (e.g., Orbitrap) to differentiate isomers by exact mass.
- Derivatizing bile acids with methyl ester or acyl-migration inhibitors to stabilize analytes prior to LC-MS .
Q. Contradiction Analysis
Q. Why do some studies report divergent effects of CDCA-d5 on cholesterol homeostasis?
Discrepancies may stem from model-specific factors:
- In vitro systems (e.g., hepatocyte lines) lack enterohepatic circulation, overemphasizing direct FXR activation.
- In vivo studies in high-fat diet models may conflate CDCA-d5’s cholesterol-lowering effects with dietary confounding.
To reconcile, use CDCA-d5 in bile duct-cannulated animals to measure biliary cholesterol excretion directly, paired with isotopic dilution assays .
Q. Methodological Tables
Table 1. Key Parameters for CDCA-d5 in Metabolic Studies
Table 2. Applications of CDCA-d5 in Disease Models
Disease Model | Application | Key Insight |
---|---|---|
NAFLD/NASH | Quantify hepatic FXR activation | CDCA-d5 reduces lipotoxicity via FXR-SHP |
Cerebrotendinous Xanthomatosis (CTX) | Track cholestanol synthesis inhibition | CDCA-d5 normalizes plasma cholestanol |
Gut Dysbiosis | Map microbial bile acid modification | CDCA-d5 enrichment correlates with Clostridium spp. |
Properties
IUPAC Name |
(4R)-4-[(3R,5S,7R,8R,9S,10S,13R,14S,17R)-2,2,3,4,4-pentadeuterio-3,7-dihydroxy-10,13-dimethyl-1,5,6,7,8,9,11,12,14,15,16,17-dodecahydrocyclopenta[a]phenanthren-17-yl]pentanoic acid | |
---|---|---|
Source | PubChem | |
URL | https://pubchem.ncbi.nlm.nih.gov | |
Description | Data deposited in or computed by PubChem | |
InChI |
InChI=1S/C24H40O4/c1-14(4-7-21(27)28)17-5-6-18-22-19(9-11-24(17,18)3)23(2)10-8-16(25)12-15(23)13-20(22)26/h14-20,22,25-26H,4-13H2,1-3H3,(H,27,28)/t14-,15+,16-,17-,18+,19+,20-,22+,23+,24-/m1/s1/i8D2,12D2,16D | |
Source | PubChem | |
URL | https://pubchem.ncbi.nlm.nih.gov | |
Description | Data deposited in or computed by PubChem | |
InChI Key |
RUDATBOHQWOJDD-FVAYXOGXSA-N | |
Source | PubChem | |
URL | https://pubchem.ncbi.nlm.nih.gov | |
Description | Data deposited in or computed by PubChem | |
Canonical SMILES |
CC(CCC(=O)O)C1CCC2C1(CCC3C2C(CC4C3(CCC(C4)O)C)O)C | |
Source | PubChem | |
URL | https://pubchem.ncbi.nlm.nih.gov | |
Description | Data deposited in or computed by PubChem | |
Isomeric SMILES |
[2H][C@]1(C(C[C@@]2([C@H]3CC[C@]4([C@H]([C@@H]3[C@@H](C[C@@H]2C1([2H])[2H])O)CC[C@@H]4[C@H](C)CCC(=O)O)C)C)([2H])[2H])O | |
Source | PubChem | |
URL | https://pubchem.ncbi.nlm.nih.gov | |
Description | Data deposited in or computed by PubChem | |
Molecular Formula |
C24H40O4 | |
Source | PubChem | |
URL | https://pubchem.ncbi.nlm.nih.gov | |
Description | Data deposited in or computed by PubChem | |
DSSTOX Substance ID |
DTXSID90745918 | |
Record name | (4R)-4-[(3R,5S,7R,8R,9S,10S,13R,14S,17R)-2,2,3,4,4-pentadeuterio-3,7-dihydroxy-10,13-dimethyl-1,5,6,7,8,9,11,12,14,15,16,17-dodecahydrocyclopenta[a]phenanthren-17-yl]pentanoic acid | |
Source | EPA DSSTox | |
URL | https://comptox.epa.gov/dashboard/DTXSID90745918 | |
Description | DSSTox provides a high quality public chemistry resource for supporting improved predictive toxicology. | |
Molecular Weight |
397.6 g/mol | |
Source | PubChem | |
URL | https://pubchem.ncbi.nlm.nih.gov | |
Description | Data deposited in or computed by PubChem | |
CAS No. |
52840-12-7 | |
Record name | (4R)-4-[(3R,5S,7R,8R,9S,10S,13R,14S,17R)-2,2,3,4,4-pentadeuterio-3,7-dihydroxy-10,13-dimethyl-1,5,6,7,8,9,11,12,14,15,16,17-dodecahydrocyclopenta[a]phenanthren-17-yl]pentanoic acid | |
Source | EPA DSSTox | |
URL | https://comptox.epa.gov/dashboard/DTXSID90745918 | |
Description | DSSTox provides a high quality public chemistry resource for supporting improved predictive toxicology. | |
Disclaimer and Information on In-Vitro Research Products
Please be aware that all articles and product information presented on BenchChem are intended solely for informational purposes. The products available for purchase on BenchChem are specifically designed for in-vitro studies, which are conducted outside of living organisms. In-vitro studies, derived from the Latin term "in glass," involve experiments performed in controlled laboratory settings using cells or tissues. It is important to note that these products are not categorized as medicines or drugs, and they have not received approval from the FDA for the prevention, treatment, or cure of any medical condition, ailment, or disease. We must emphasize that any form of bodily introduction of these products into humans or animals is strictly prohibited by law. It is essential to adhere to these guidelines to ensure compliance with legal and ethical standards in research and experimentation.