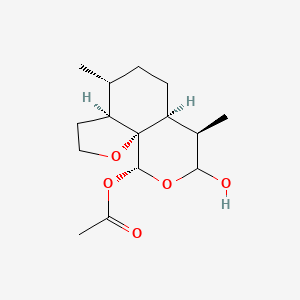
Dihydro Artemisinin Tetrahydrofuran Acetate
Overview
Description
Dihydro Artemisinin Tetrahydrofuran Acetate is a derivative of artemisinin, a natural compound extracted from the plant Artemisia annua. Artemisinin and its derivatives have been widely recognized for their potent antimalarial properties. This compound is a semi-synthetic compound that has shown promise in various scientific research applications, particularly in the fields of medicine and pharmacology .
Preparation Methods
Synthetic Routes and Reaction Conditions: The reduction of artemisinin can be achieved using sodium borohydride in the presence of boron trifluoride etherate . The tetrahydrofuran ring is introduced through a cyclization reaction, and the final acetylation step is carried out using acetic anhydride in the presence of a base such as pyridine .
Industrial Production Methods: Industrial production of Dihydro Artemisinin Tetrahydrofuran Acetate follows similar synthetic routes but on a larger scale. The process involves optimizing reaction conditions to ensure high yield and purity. The use of continuous flow reactors and advanced purification techniques such as high-performance liquid chromatography (HPLC) are common in industrial settings .
Chemical Reactions Analysis
Types of Reactions: Dihydro Artemisinin Tetrahydrofuran Acetate undergoes various chemical reactions, including:
Oxidation: The compound can be oxidized to form different derivatives, which may have distinct pharmacological properties.
Reduction: Reduction reactions can further modify the compound, potentially enhancing its biological activity.
Substitution: Substitution reactions, particularly at the acetate group, can lead to the formation of new derivatives with altered properties.
Common Reagents and Conditions:
Oxidation: Common oxidizing agents include hydrogen peroxide and potassium permanganate.
Reduction: Sodium borohydride and lithium aluminum hydride are frequently used reducing agents.
Substitution: Acetic anhydride and various bases such as pyridine are used for acetylation reactions.
Major Products Formed: The major products formed from these reactions include various artemisinin derivatives, each with unique pharmacological profiles. These derivatives are often evaluated for their antimalarial, anti-inflammatory, and anticancer activities .
Scientific Research Applications
Dihydro Artemisinin Tetrahydrofuran Acetate has a wide range of scientific research applications:
Chemistry: The compound is used as a starting material for the synthesis of new artemisinin derivatives with potential therapeutic applications.
Mechanism of Action
The mechanism of action of Dihydro Artemisinin Tetrahydrofuran Acetate involves the generation of reactive oxygen species (ROS) that cause oxidative stress in target cells. This oxidative stress leads to the disruption of cellular functions and ultimately cell death. The compound targets various molecular pathways, including the induction of apoptosis through the activation of caspases and the modulation of inflammatory cytokines .
Comparison with Similar Compounds
Artemisinin: The parent compound from which Dihydro Artemisinin Tetrahydrofuran Acetate is derived.
Dihydroartemisinin: A direct derivative of artemisinin with potent antimalarial activity.
Artemether: A methyl ether derivative of dihydroartemisinin used in combination therapies for malaria.
Artesunate: A water-soluble derivative of dihydroartemisinin used for severe malaria.
Uniqueness: this compound is unique due to its modified structure, which includes a tetrahydrofuran ring and an acetate group. These modifications enhance its pharmacokinetic properties and potentially broaden its therapeutic applications compared to other artemisinin derivatives .
Biological Activity
Dihydro Artemisinin Tetrahydrofuran Acetate (DHTA) is a derivative of dihydroartemisinin (DHA), which is known for its potent antimalarial and anticancer properties. This article explores the biological activity of DHTA, focusing on its mechanisms of action, therapeutic applications, and recent research findings.
DHTA, like other artemisinin derivatives, exerts its biological effects primarily through the generation of free radicals upon activation by iron. These radicals can alkylate various biological targets, leading to cellular damage and apoptosis in parasitic and cancerous cells. The proposed mechanisms include:
- Iron-Catalyzed Activation: DHTA interacts with iron to produce reactive oxygen species (ROS), which are responsible for inducing oxidative stress in target cells. This process is crucial for its antimalarial activity, as it disrupts the malaria parasite's cellular functions .
- Alkylation of Cellular Targets: The free radicals generated from DHTA can alkylate proteins and lipids within the cell, leading to cell death. This mechanism is particularly significant in cancer cells, where it can trigger apoptosis or other forms of cell death .
Antimalarial Activity
DHTA has shown significant efficacy against malaria parasites. Studies have demonstrated that it can effectively inhibit the growth of Plasmodium falciparum, the causative agent of malaria, through the following actions:
- Inhibition of Heme Detoxification: DHTA disrupts the detoxification of heme in malaria parasites, leading to increased oxidative stress and eventual cell death .
- Synergistic Effects with Other Antimalarials: When combined with other antimalarial drugs, DHTA has been shown to enhance therapeutic outcomes, reducing the likelihood of resistance development .
Anticancer Activity
Recent research highlights the potential of DHTA as an anticancer agent. Its ability to induce apoptosis in various cancer cell lines has been documented:
- Cell Lines Affected: DHTA exhibits antiproliferative effects on breast, colon, pancreas, liver, lung, ovarian, and prostate cancer cells .
- Mechanistic Insights: The compound has been found to reduce c-MYC protein levels in tumor cells, leading to cell cycle arrest and apoptosis. This suggests a multi-targeted approach in its anticancer activity .
Case Studies and Research Findings
Several studies have explored the biological activity of DHTA and its derivatives:
Q & A
Basic Research Questions
Q. What are the primary methodologies for synthesizing DATHA, and how do reaction conditions influence yield?
DATHA synthesis typically involves esterification of dihydroartemisinin with tetrahydrofuran acetate derivatives under controlled conditions. Solvent selection (e.g., tetrahydrofuran (THF) or ethyl acetate) and catalysts (e.g., acidic or enzymatic) are critical. For example, THF acts as both a solvent and reactant in some protocols, with yields optimized by adjusting temperature (15–25°C) and reaction time (3–16 hours) . Post-synthesis purification often employs gradient elution in reversed-phase chromatography, using mobile phases containing THF and sodium acetate buffers .
Q. How can solubility parameters of DATHA be experimentally determined for formulation studies?
Solubility in organic solvents (e.g., THF, ethyl acetate) is quantified via thermodynamic measurements, including density, viscosity, and speed of sound in binary mixtures. The Redlich–Kister equation and semiempirical models are used to correlate experimental data, enabling predictions of DATHA’s miscibility and phase behavior . For aqueous solubility, co-solvency approaches with THF/water mixtures (e.g., 75:25 v/v) are common, leveraging THF’s ability to solubilize hydrophobic moieties while maintaining stability .
Q. What analytical techniques are recommended for quantifying DATHA purity and degradation products?
Ultra-performance liquid chromatography (UPLC) with fluorescence detection is widely used. Mobile phases often combine THF (2.5%), methanol (20–80%), and sodium acetate buffers (pH 5.3) to achieve baseline separation of DATHA from impurities. Derivatization with o-phthalaldehyde (OPA) enhances detection sensitivity for low-concentration samples . For structural confirmation, NMR (e.g., DMSO-d6 as solvent) and mass spectrometry are employed, with protocols optimized to avoid THF-induced signal interference .
Advanced Research Questions
Q. How can process optimization improve DATHA synthesis scalability while minimizing THF waste?
Triple-column extractive distillation is a promising method for THF recovery and reuse in large-scale synthesis. This involves computer-aided molecular design (CAMD) to select optimal entrainers (e.g., ionic liquids) that enhance separation efficiency. Thermodynamic analysis (e.g., vapor-liquid equilibrium modeling) and heat integration reduce energy consumption by up to 30% compared to conventional methods . Process parameters (e.g., reflux ratio, feed stage location) are optimized using Aspen Plus simulations .
Q. What experimental designs are suitable for studying DATHA’s stability under oxidative stress?
Accelerated stability studies involve exposing DATHA to peroxyl radicals or UV light in THF/water mixtures. Oxidation products (e.g., hydroxylated derivatives) are monitored via UPLC with fluorescence detection (λex 340 nm, λem 440 nm). Kinetic modeling of degradation rates under varying pH (4.0–6.3) and temperature (25–40°C) conditions helps identify degradation pathways . Data normalization to control samples (e.g., unoxidized DATHA) ensures reproducibility .
Q. How do molecular interactions between DATHA and polymeric matrices affect drug delivery systems?
Molecular dynamics (MD) simulations reveal that DATHA’s tetrahydrofuran acetate group forms hydrogen bonds with poly(methyl methacrylate) (PMMA) matrices, enhancing encapsulation efficiency. Experimental validation involves preparing PMMA/DATHA nanocomposites via solvent casting (THF as solvent) and characterizing interactions using FTIR (C=O stretching shifts) and DSC (glass transition temperature changes) . Solubility parameters (e.g., Hansen solubility coefficients) predict compatibility between DATHA and polymer excipients .
Properties
IUPAC Name |
[(3aS,4R,6aS,7R,10R,10aR)-8-hydroxy-4,7-dimethyl-2,3,3a,4,5,6,6a,7,8,10-decahydrofuro[3,2-i]isochromen-10-yl] acetate | |
---|---|---|
Source | PubChem | |
URL | https://pubchem.ncbi.nlm.nih.gov | |
Description | Data deposited in or computed by PubChem | |
InChI |
InChI=1S/C15H24O5/c1-8-4-5-12-9(2)13(17)20-14(19-10(3)16)15(12)11(8)6-7-18-15/h8-9,11-14,17H,4-7H2,1-3H3/t8-,9-,11+,12+,13?,14+,15-/m1/s1 | |
Source | PubChem | |
URL | https://pubchem.ncbi.nlm.nih.gov | |
Description | Data deposited in or computed by PubChem | |
InChI Key |
MXTIBMLCXHSHGJ-AAAPMNIRSA-N | |
Source | PubChem | |
URL | https://pubchem.ncbi.nlm.nih.gov | |
Description | Data deposited in or computed by PubChem | |
Canonical SMILES |
CC1CCC2C(C(OC(C23C1CCO3)OC(=O)C)O)C | |
Source | PubChem | |
URL | https://pubchem.ncbi.nlm.nih.gov | |
Description | Data deposited in or computed by PubChem | |
Isomeric SMILES |
C[C@@H]1CC[C@H]2[C@H](C(O[C@@H]([C@@]23[C@H]1CCO3)OC(=O)C)O)C | |
Source | PubChem | |
URL | https://pubchem.ncbi.nlm.nih.gov | |
Description | Data deposited in or computed by PubChem | |
Molecular Formula |
C15H24O5 | |
Source | PubChem | |
URL | https://pubchem.ncbi.nlm.nih.gov | |
Description | Data deposited in or computed by PubChem | |
DSSTOX Substance ID |
DTXSID60747641 | |
Record name | (3aS,4R,6aS,7R,10R,10aR)-8-Hydroxy-4,7-dimethyloctahydro-2H,10H-furo[3,2-i][2]benzopyran-10-yl acetate | |
Source | EPA DSSTox | |
URL | https://comptox.epa.gov/dashboard/DTXSID60747641 | |
Description | DSSTox provides a high quality public chemistry resource for supporting improved predictive toxicology. | |
Molecular Weight |
284.35 g/mol | |
Source | PubChem | |
URL | https://pubchem.ncbi.nlm.nih.gov | |
Description | Data deposited in or computed by PubChem | |
CAS No. |
198817-95-7 | |
Record name | (3aS,4R,6aS,7R,10R,10aR)-8-Hydroxy-4,7-dimethyloctahydro-2H,10H-furo[3,2-i][2]benzopyran-10-yl acetate | |
Source | EPA DSSTox | |
URL | https://comptox.epa.gov/dashboard/DTXSID60747641 | |
Description | DSSTox provides a high quality public chemistry resource for supporting improved predictive toxicology. | |
Disclaimer and Information on In-Vitro Research Products
Please be aware that all articles and product information presented on BenchChem are intended solely for informational purposes. The products available for purchase on BenchChem are specifically designed for in-vitro studies, which are conducted outside of living organisms. In-vitro studies, derived from the Latin term "in glass," involve experiments performed in controlled laboratory settings using cells or tissues. It is important to note that these products are not categorized as medicines or drugs, and they have not received approval from the FDA for the prevention, treatment, or cure of any medical condition, ailment, or disease. We must emphasize that any form of bodily introduction of these products into humans or animals is strictly prohibited by law. It is essential to adhere to these guidelines to ensure compliance with legal and ethical standards in research and experimentation.