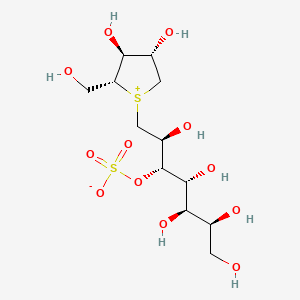
Kotalanol
Overview
Description
Kotalanol is a potent natural α-glucosidase inhibitor isolated from the roots and stems of the plant Salacia reticulata, which is traditionally used in Ayurvedic medicine for the treatment of diabetes. This compound has a unique thiosugar sulfonium sulfate structure and exhibits significant antidiabetic properties by inhibiting the enzyme α-glucosidase, which is involved in the breakdown of carbohydrates into glucose .
Preparation Methods
Synthetic Routes and Reaction Conditions: The synthesis of kotalanol involves several steps, starting from readily available sugars. One of the key steps includes the formation of a thiosugar sulfonium intermediate, which is then converted into this compound through a series of chemical reactions. The synthetic route typically involves the use of protecting groups to ensure the selective formation of the desired product .
Industrial Production Methods: Industrial production of this compound is still under development, with research focusing on optimizing the yield and purity of the compound. The process involves the extraction of this compound from the plant Salacia reticulata, followed by purification using chromatographic techniques. Advances in biotechnological methods are also being explored to enhance the production efficiency .
Chemical Reactions Analysis
Types of Reactions: Kotalanol undergoes various chemical reactions, including:
Oxidation: this compound can be oxidized to form sulfoxides and sulfones.
Reduction: Reduction reactions can convert this compound into its corresponding thiol derivatives.
Substitution: this compound can undergo nucleophilic substitution reactions, where the sulfonium group is replaced by other nucleophiles.
Common Reagents and Conditions:
Oxidation: Common oxidizing agents include hydrogen peroxide and peracids.
Reduction: Reducing agents such as sodium borohydride and lithium aluminum hydride are used.
Substitution: Nucleophiles like thiols, amines, and halides are employed under mild to moderate conditions.
Major Products: The major products formed from these reactions include sulfoxides, sulfones, thiol derivatives, and substituted this compound analogs .
Scientific Research Applications
Kotalanol has a wide range of scientific research applications, including:
Chemistry: Used as a model compound for studying thiosugar chemistry and sulfonium ion reactivity.
Biology: Investigated for its role in inhibiting carbohydrate-hydrolyzing enzymes and its potential as a dietary supplement for managing blood sugar levels.
Medicine: Explored for its antidiabetic properties and potential use in developing new therapeutic agents for diabetes management.
Mechanism of Action
Kotalanol exerts its effects by inhibiting the enzyme α-glucosidase, which is responsible for breaking down carbohydrates into glucose in the small intestine. By inhibiting this enzyme, this compound reduces the rate of glucose absorption, thereby lowering postprandial blood glucose levels. The molecular target of this compound is the active site of α-glucosidase, where it binds and prevents the enzyme from catalyzing the hydrolysis of carbohydrates .
Comparison with Similar Compounds
Kotalanol is unique among α-glucosidase inhibitors due to its thiosugar sulfonium sulfate structure. Similar compounds include:
Salacinol: Another potent α-glucosidase inhibitor isolated from Salacia reticulata, with a similar mechanism of action but different structural features.
Neothis compound: A related compound with a slightly different thiosugar structure, exhibiting similar antidiabetic properties.
Acarbose: A clinically used α-glucosidase inhibitor with a different chemical structure but similar therapeutic effects
This compound stands out due to its higher potency and unique structural features, making it a valuable compound for further research and development in the field of diabetes management .
Biological Activity
Kotalanol, a naturally occurring compound isolated from the roots and stems of Salacia reticulata, has garnered significant attention due to its potent biological activities, particularly as an α-glucosidase inhibitor. This article delves into the biological activity of this compound, examining its mechanisms, efficacy, and potential therapeutic applications, supported by data tables and case studies.
This compound is characterized as a sulfonium-ion thiosugar. Its structure comprises a 1-deoxyheptosyl-3-sulfate anion and a 1-deoxy-4-thio-D-arabinofuranosyl sulfonium cation. The compound exhibits strong inhibitory activity against α-glucosidases, enzymes responsible for carbohydrate metabolism, thereby influencing glucose absorption in the intestines.
Inhibition Mechanism
This compound's mechanism involves competitive inhibition of α-glucosidase, which prevents the hydrolysis of oligosaccharides into monosaccharides. This action helps regulate postprandial blood glucose levels, making this compound a candidate for managing diabetes.
Efficacy as an α-Glucosidase Inhibitor
Research indicates that this compound displays more potent inhibitory effects against sucrase compared to other known inhibitors like salacinol and acarbose. In vitro studies have demonstrated that this compound can significantly reduce the activity of various α-glucosidases.
Comparative Inhibition Potency
Compound | Inhibition Activity (IC50) | Source |
---|---|---|
This compound | 0.5 µM | Salacia reticulata |
Salacinol | 0.7 µM | Salacia reticulata |
Acarbose | 2.0 µM | Synthetic drug |
1. Antidiabetic Effects
A study conducted on diabetic rats showed that oral administration of this compound significantly lowered blood glucose levels compared to control groups. The results indicate that this compound not only inhibits α-glucosidase but also improves overall glycemic control.
2. Antiviral Activity
This compound has also been evaluated for its antiviral properties. In a study involving H1N1-infected mice, extracts from Salacia reticulata, rich in this compound, resulted in a significant reduction in cough incidence and pulmonary inflammation when administered at a dosage of 0.6 mg/day .
Structural Studies and Binding Affinity
Homology modeling studies have provided insights into the binding affinity of this compound with human α-glucosidases. Docking simulations revealed that this compound forms multiple hydrogen bonds with key amino acid residues within the enzyme's active site, enhancing its inhibitory potency .
Future Directions in Research
The ongoing exploration of this compound includes:
- Structural Modifications : Developing analogues to enhance efficacy and reduce side effects.
- Clinical Trials : Evaluating long-term safety and effectiveness in human subjects.
- Mechanistic Studies : Further elucidating the molecular pathways influenced by this compound.
Q & A
Basic Research Questions
Q. How was the stereochemical structure of kotalanol confirmed, and what synthetic strategies were pivotal in resolving ambiguities?
this compound's structure was determined through synthesis and comparative analysis of physical data with natural isolates. Pinto et al. synthesized this compound from D-perseitol, a heptitol derived from avocado plants, using cyclic sulfates and PMB-protected 4-thio-D-arabinitol. The stereochemistry at C20 (S-configuration), C40 (R-configuration), and C50 (S-configuration) was critical for α-glucosidase inhibitory activity. Structural confirmation relied on matching synthesized de-O-sulfonated this compound epimers (267 and 268) with natural derivatives via NMR and crystallography .
Q. What methodologies are recommended for evaluating this compound's α-glucosidase inhibitory activity in vitro?
Standard assays involve recombinant human maltase-glucoamylase (ntMGAM) and sucrase-isomaltase (ntSI). Inhibitory potency (IC₅₀) is measured using fluorogenic substrates (e.g., 4-methylumbelliferyl-α-D-glucopyranoside). For example, this compound exhibits IC₅₀ values of 3.9–4.9 μM against human maltase, comparable to acarbose. Stability in artificial gastric juice and intestinal absorption are assessed via in-situ rat-ligated intestinal loop models .
Q. How does this compound's stability profile influence its pharmacological potential?
this compound shows high stability in gastric environments (pH 1.2) and minimal intestinal absorption, making it a non-systemic inhibitor. This localized action reduces systemic side effects, a key advantage over synthetic inhibitors like acarbose. In vivo studies confirm its retention in the intestinal lumen, where it competitively inhibits carbohydrate hydrolysis .
Advanced Research Questions
Q. What structural features of this compound analogues enhance ntMGAM/ntSI selectivity?
Modifications at the C-5' sulfate group and heteroatom substitution (e.g., nitrogen or selenium) significantly alter selectivity. For instance:
- C-5' diastereomers of de-O-sulfonated this compound (110) : ntMGAM inhibition = 0.026 ± 0.02 μM.
- Selenium analogue (115) : ntMGAM inhibition = 0.02 ± 0.03 μM (10x more potent than nitrogen analogues). Trp327 in ntSI displaces the sulfate group by 1–1.5 Å, reducing hydrogen bonding with Thr205 compared to ntMGAM, explaining domain-specific selectivity .
Q. How do homology modeling and docking studies elucidate this compound's binding mechanisms?
Homology models of CtMGAM and CtSI were built using ntMGAM-kotalanol complexes (PDB: 3L4V). This compound forms hydrogen bonds with Asp443, Arg672, and Asp542 in ntMGAM, while ntSI interactions involve Trp327 and Asp231. Molecular dynamics reveal deeper binding pockets in CtMGAM, enhancing van der Waals interactions with hydrophobic derivatives .
Q. What contradictions exist in reported inhibitory data for this compound analogues, and how can they be resolved?
Discrepancies arise from assay conditions (e.g., substrate specificity, enzyme sources). For example:
- Selenium analogue (112) : ntMGAM IC₅₀ = 0.08 ± 0.06 μM.
- Diastereomer (113) : IC₅₀ = 7.2 ± 0.7 nM (100x variation). Standardizing recombinant enzyme batches and pre-incubation times (≥30 min) minimizes variability. Cross-validation with X-ray co-crystallography resolves ambiguous binding modes .
Q. How does de-O-sulfonation impact this compound's bioactivity and synthetic feasibility?
De-O-sulfonated this compound (114, 115) retains potency (ntMGAM IC₅₀ = 0.061 μM) but simplifies synthesis by eliminating sulfate protection/deprotection steps. However, sulfonation at C-5 is critical for water solubility and charge-mediated enzyme interactions. Synthetic routes using benzylidene acetals and PMB protection improve yields but require rigorous stereocontrol .
Q. What role does the sulfonium ion play in this compound's mechanism compared to salacinol?
The sulfonium ion in this compound stabilizes transition states via electrostatic interactions with Asp443 (ntMGAM). Unlike salacinol, this compound's extended heptitol chain occupies the +1 subsite, enhancing affinity. Mutagenesis studies show Thr205 mutations in ntMGAM reduce this compound's inhibition by 60%, highlighting residue-specific binding .
Q. Methodological Guidelines
- For SAR Studies : Prioritize C-5' sulfonation and heteroatom substitution (Se > N) to optimize ntMGAM selectivity. Use in silico docking (MOE, AutoDock) with experimental structures (PDB: 3L4V, 3TOP) .
- For Structural Analysis : Combine NMR (¹H, ¹³C, HSQC) with X-ray crystallography to resolve stereochemical ambiguities. Reference synthesized epimers (267, 268) as controls .
- For Data Reproducibility : Report enzyme sources (recombinant vs. tissue-derived), pre-incubation times, and substrate concentrations. Use artificial gastric juice (pH 1.2, pepsin) for stability assays .
Properties
IUPAC Name |
[(2S,3S,4R,5R,6S)-1-[(2R,3S,4S)-3,4-dihydroxy-2-(hydroxymethyl)thiolan-1-ium-1-yl]-2,4,5,6,7-pentahydroxyheptan-3-yl] sulfate | |
---|---|---|
Source | PubChem | |
URL | https://pubchem.ncbi.nlm.nih.gov | |
Description | Data deposited in or computed by PubChem | |
InChI |
InChI=1S/C12H24O12S2/c13-1-5(15)10(19)11(20)12(24-26(21,22)23)7(17)4-25-3-6(16)9(18)8(25)2-14/h5-20H,1-4H2/t5-,6+,7+,8+,9-,10+,11+,12+,25?/m0/s1 | |
Source | PubChem | |
URL | https://pubchem.ncbi.nlm.nih.gov | |
Description | Data deposited in or computed by PubChem | |
InChI Key |
OMKXVFDVAGCPBS-GTEYUELZSA-N | |
Source | PubChem | |
URL | https://pubchem.ncbi.nlm.nih.gov | |
Description | Data deposited in or computed by PubChem | |
Canonical SMILES |
C1C(C(C([S+]1CC(C(C(C(C(CO)O)O)O)OS(=O)(=O)[O-])O)CO)O)O | |
Source | PubChem | |
URL | https://pubchem.ncbi.nlm.nih.gov | |
Description | Data deposited in or computed by PubChem | |
Isomeric SMILES |
C1[C@H]([C@@H]([C@H]([S+]1C[C@H]([C@H]([C@@H]([C@@H]([C@H](CO)O)O)O)OS(=O)(=O)[O-])O)CO)O)O | |
Source | PubChem | |
URL | https://pubchem.ncbi.nlm.nih.gov | |
Description | Data deposited in or computed by PubChem | |
Molecular Formula |
C12H24O12S2 | |
Source | PubChem | |
URL | https://pubchem.ncbi.nlm.nih.gov | |
Description | Data deposited in or computed by PubChem | |
DSSTOX Substance ID |
DTXSID10655282 | |
Record name | (2S,3S,4R,5R,6S)-1-[(2R,3S,4S)-3,4-Dihydroxy-2-(hydroxymethyl)thiolan-1-ium-1-yl]-2,4,5,6,7-pentahydroxyheptan-3-yl sulfate (non-preferred name) | |
Source | EPA DSSTox | |
URL | https://comptox.epa.gov/dashboard/DTXSID10655282 | |
Description | DSSTox provides a high quality public chemistry resource for supporting improved predictive toxicology. | |
Molecular Weight |
424.4 g/mol | |
Source | PubChem | |
URL | https://pubchem.ncbi.nlm.nih.gov | |
Description | Data deposited in or computed by PubChem | |
CAS No. |
214491-07-3 | |
Record name | (2S,3S,4R,5R,6S)-1-[(2R,3S,4S)-3,4-Dihydroxy-2-(hydroxymethyl)thiolan-1-ium-1-yl]-2,4,5,6,7-pentahydroxyheptan-3-yl sulfate (non-preferred name) | |
Source | EPA DSSTox | |
URL | https://comptox.epa.gov/dashboard/DTXSID10655282 | |
Description | DSSTox provides a high quality public chemistry resource for supporting improved predictive toxicology. | |
Disclaimer and Information on In-Vitro Research Products
Please be aware that all articles and product information presented on BenchChem are intended solely for informational purposes. The products available for purchase on BenchChem are specifically designed for in-vitro studies, which are conducted outside of living organisms. In-vitro studies, derived from the Latin term "in glass," involve experiments performed in controlled laboratory settings using cells or tissues. It is important to note that these products are not categorized as medicines or drugs, and they have not received approval from the FDA for the prevention, treatment, or cure of any medical condition, ailment, or disease. We must emphasize that any form of bodily introduction of these products into humans or animals is strictly prohibited by law. It is essential to adhere to these guidelines to ensure compliance with legal and ethical standards in research and experimentation.