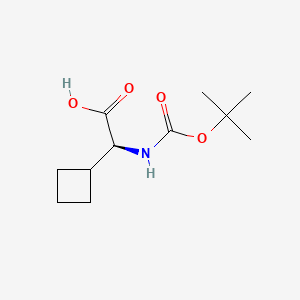
(S)-2-((tert-Butoxycarbonyl)amino)-2-cyclobutylacetic acid
Overview
Description
Mechanism of Action
Target of Action
It’s known that this compound is a glycine derivative and can be used for pi3k inhibitor synthesis . PI3Ks (Phosphoinositide 3-kinases) are a family of enzymes involved in cellular functions such as cell growth, proliferation, differentiation, motility, survival and intracellular trafficking, which in turn are involved in cancer.
Mode of Action
The compound is a boc-protected amino acid . Boc-protection is a common method used in organic chemistry to protect amines, particularly in peptide synthesis . The Boc group is stable towards most nucleophiles and bases, allowing for selective reactions .
Biochemical Pathways
As a potential pi3k inhibitor, it may affect the pi3k/akt/mtor pathway, which is critical in many aspects of cell growth and survival .
Result of Action
As a potential pi3k inhibitor, it could potentially inhibit cell growth and proliferation, induce cell cycle arrest, and promote apoptosis .
Action Environment
Like most chemicals, its stability and reactivity may be influenced by factors such as temperature, ph, and the presence of other chemicals .
Preparation Methods
Synthetic Routes and Reaction Conditions
The synthesis of Boc-L-cyclobutylglycine involves a multi-step reaction process. One of the common synthetic routes includes the following steps :
n-Butyllithium (n-BuLi) in tetrahydrofuran (THF): The reaction is initiated at -78°C for 15 minutes, followed by a gradual increase in temperature to -10°C.
n-Butyllithium in THF: The reaction continues at -40°C.
Sodium amalgam (Na-Hg) and sodium phosphate (Na2HPO4) in methanol: This step is carried out for 3 hours at temperatures ranging from 0 to 20°C.
Pyridinium p-toluenesulfonate (PPTS) in ethanol: The reaction is conducted for 6 hours at 50°C.
Chromium trioxide (CrO3) and sulfuric acid (H2SO4): The final step involves a 1-hour reaction at temperatures between 0 and 20°C.
Industrial Production Methods
Industrial production methods for Boc-L-cyclobutylglycine are not extensively documented. the synthesis typically follows similar multi-step processes as described above, with optimizations for large-scale production.
Chemical Reactions Analysis
Types of Reactions
Boc-L-cyclobutylglycine undergoes various chemical reactions, including:
Oxidation: The compound can be oxidized using reagents like chromium trioxide.
Reduction: Reduction reactions can be performed using sodium amalgam.
Substitution: Substitution reactions often involve the use of n-butyllithium.
Common Reagents and Conditions
Oxidation: Chromium trioxide (CrO3) and sulfuric acid (H2SO4).
Reduction: Sodium amalgam (Na-Hg) and sodium phosphate (Na2HPO4) in methanol.
Substitution: n-Butyllithium (n-BuLi) in tetrahydrofuran (THF).
Major Products Formed
The major products formed from these reactions depend on the specific conditions and reagents used. For example, oxidation with chromium trioxide typically results in the formation of carboxylic acids.
Scientific Research Applications
Boc-L-cyclobutylglycine is widely used in scientific research, particularly in the synthesis of PI3K inhibitors . These inhibitors are crucial in the study of various biological processes and diseases, including cancer, diabetes, and inflammatory conditions. The compound is also used in the development of novel therapeutic agents and in the study of cellular signaling pathways .
Comparison with Similar Compounds
Boc-L-cyclobutylglycine is unique due to its specific structure and its role in PI3K inhibitor synthesis. Similar compounds include other glycine derivatives used in the synthesis of kinase inhibitors, such as:
- Boc-L-alanine
- Boc-L-valine
- Boc-L-leucine
These compounds share similar protective groups but differ in their side chains, which can influence their reactivity and applications .
Properties
IUPAC Name |
(2S)-2-cyclobutyl-2-[(2-methylpropan-2-yl)oxycarbonylamino]acetic acid | |
---|---|---|
Source | PubChem | |
URL | https://pubchem.ncbi.nlm.nih.gov | |
Description | Data deposited in or computed by PubChem | |
InChI |
InChI=1S/C11H19NO4/c1-11(2,3)16-10(15)12-8(9(13)14)7-5-4-6-7/h7-8H,4-6H2,1-3H3,(H,12,15)(H,13,14)/t8-/m0/s1 | |
Source | PubChem | |
URL | https://pubchem.ncbi.nlm.nih.gov | |
Description | Data deposited in or computed by PubChem | |
InChI Key |
OHDFGIXTRBDGRB-QMMMGPOBSA-N | |
Source | PubChem | |
URL | https://pubchem.ncbi.nlm.nih.gov | |
Description | Data deposited in or computed by PubChem | |
Canonical SMILES |
CC(C)(C)OC(=O)NC(C1CCC1)C(=O)O | |
Source | PubChem | |
URL | https://pubchem.ncbi.nlm.nih.gov | |
Description | Data deposited in or computed by PubChem | |
Isomeric SMILES |
CC(C)(C)OC(=O)N[C@@H](C1CCC1)C(=O)O | |
Source | PubChem | |
URL | https://pubchem.ncbi.nlm.nih.gov | |
Description | Data deposited in or computed by PubChem | |
Molecular Formula |
C11H19NO4 | |
Source | PubChem | |
URL | https://pubchem.ncbi.nlm.nih.gov | |
Description | Data deposited in or computed by PubChem | |
DSSTOX Substance ID |
DTXSID201164281 | |
Record name | (αS)-α-[[(1,1-Dimethylethoxy)carbonyl]amino]cyclobutaneacetic acid | |
Source | EPA DSSTox | |
URL | https://comptox.epa.gov/dashboard/DTXSID201164281 | |
Description | DSSTox provides a high quality public chemistry resource for supporting improved predictive toxicology. | |
Molecular Weight |
229.27 g/mol | |
Source | PubChem | |
URL | https://pubchem.ncbi.nlm.nih.gov | |
Description | Data deposited in or computed by PubChem | |
CAS No. |
155905-77-4 | |
Record name | (αS)-α-[[(1,1-Dimethylethoxy)carbonyl]amino]cyclobutaneacetic acid | |
Source | CAS Common Chemistry | |
URL | https://commonchemistry.cas.org/detail?cas_rn=155905-77-4 | |
Description | CAS Common Chemistry is an open community resource for accessing chemical information. Nearly 500,000 chemical substances from CAS REGISTRY cover areas of community interest, including common and frequently regulated chemicals, and those relevant to high school and undergraduate chemistry classes. This chemical information, curated by our expert scientists, is provided in alignment with our mission as a division of the American Chemical Society. | |
Explanation | The data from CAS Common Chemistry is provided under a CC-BY-NC 4.0 license, unless otherwise stated. | |
Record name | (αS)-α-[[(1,1-Dimethylethoxy)carbonyl]amino]cyclobutaneacetic acid | |
Source | EPA DSSTox | |
URL | https://comptox.epa.gov/dashboard/DTXSID201164281 | |
Description | DSSTox provides a high quality public chemistry resource for supporting improved predictive toxicology. | |
Disclaimer and Information on In-Vitro Research Products
Please be aware that all articles and product information presented on BenchChem are intended solely for informational purposes. The products available for purchase on BenchChem are specifically designed for in-vitro studies, which are conducted outside of living organisms. In-vitro studies, derived from the Latin term "in glass," involve experiments performed in controlled laboratory settings using cells or tissues. It is important to note that these products are not categorized as medicines or drugs, and they have not received approval from the FDA for the prevention, treatment, or cure of any medical condition, ailment, or disease. We must emphasize that any form of bodily introduction of these products into humans or animals is strictly prohibited by law. It is essential to adhere to these guidelines to ensure compliance with legal and ethical standards in research and experimentation.