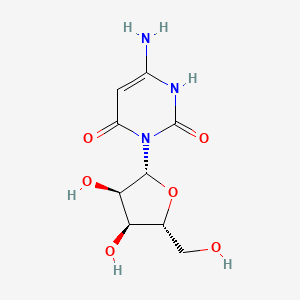
6-Oxocytidine
Overview
Description
6-Oxocytidine is a modified nucleoside analogue known for its unique structural properties and potential applications in various scientific fields. It is a derivative of cytidine, where the oxygen atom is introduced at the sixth position of the pyrimidine ring. This modification imparts distinct chemical and biological characteristics to the compound, making it a subject of interest in nucleic acid research and drug development .
Preparation Methods
Synthetic Routes and Reaction Conditions: The synthesis of 6-oxocytidine involves the incorporation of an oxygen atom at the sixth position of the cytidine molecule. One common method includes the use of 2′-O-methyl-3′-O-phosphoramidite building blocks. These building blocks are synthesized and incorporated into oligodeoxynucleotides via phosphoramidite chemistry . The reaction conditions typically involve the use of sodium cacodylate buffer, sodium chloride, and magnesium chloride to facilitate the formation of the desired product .
Industrial Production Methods: While specific industrial production methods for this compound are not extensively documented, the general approach would involve large-scale synthesis using similar phosphoramidite chemistry techniques. Optimization of reaction conditions and purification processes would be essential to achieve high yields and purity suitable for industrial applications.
Chemical Reactions Analysis
Types of Reactions: 6-Oxocytidine undergoes various chemical reactions, including:
Substitution: The compound can participate in substitution reactions where functional groups are replaced by other atoms or groups.
Common Reagents and Conditions:
Oxidation: Common oxidizing agents such as hydrogen peroxide or potassium permanganate can be used.
Substitution: Reagents like halogens or nucleophiles can facilitate substitution reactions.
Major Products: The major products formed from these reactions depend on the specific reagents and conditions used. For example, oxidation may yield various oxidized derivatives, while substitution reactions can produce a range of substituted nucleoside analogues.
Scientific Research Applications
6-Oxocytidine has several applications in scientific research, including:
Chemistry: It is used in the study of nucleic acid structures and interactions.
Biology: The compound is utilized in the development of nucleic acid-based probes and sensors. Its ability to form stable complexes with complementary strands makes it useful in molecular biology experiments.
Medicine: this compound is explored for its potential therapeutic applications, particularly in antiviral and anticancer research. Its modified structure can enhance the efficacy and specificity of nucleoside-based drugs.
Industry: The compound’s stability and reactivity make it suitable for use in various industrial processes, including the synthesis of nucleic acid analogues and the development of diagnostic tools.
Mechanism of Action
The mechanism of action of 6-oxocytidine involves its incorporation into nucleic acids, where it can form stable complexes with complementary strands. This stability is attributed to the unique hydrogen bonding patterns facilitated by the oxygen atom at the sixth position . The compound can interact with specific molecular targets, such as enzymes involved in nucleic acid metabolism, thereby influencing various biological pathways.
Comparison with Similar Compounds
Cytidine: The parent compound of 6-oxocytidine, lacking the oxygen modification.
Uridine: Another nucleoside analogue with a similar structure but different functional groups.
6-Aminouracil: A related compound used in the synthesis of nucleoside analogues.
Uniqueness: The oxygen modification at the sixth position imparts distinct chemical and biological properties that differentiate it from other nucleoside analogues .
Properties
IUPAC Name |
6-amino-3-[(2R,3R,4S,5R)-3,4-dihydroxy-5-(hydroxymethyl)oxolan-2-yl]-1H-pyrimidine-2,4-dione | |
---|---|---|
Source | PubChem | |
URL | https://pubchem.ncbi.nlm.nih.gov | |
Description | Data deposited in or computed by PubChem | |
InChI |
InChI=1S/C9H13N3O6/c10-4-1-5(14)12(9(17)11-4)8-7(16)6(15)3(2-13)18-8/h1,3,6-8,13,15-16H,2,10H2,(H,11,17)/t3-,6-,7-,8-/m1/s1 | |
Source | PubChem | |
URL | https://pubchem.ncbi.nlm.nih.gov | |
Description | Data deposited in or computed by PubChem | |
InChI Key |
ROTWXLHBETVAFW-YXZULKJRSA-N | |
Source | PubChem | |
URL | https://pubchem.ncbi.nlm.nih.gov | |
Description | Data deposited in or computed by PubChem | |
Canonical SMILES |
C1=C(NC(=O)N(C1=O)C2C(C(C(O2)CO)O)O)N | |
Source | PubChem | |
URL | https://pubchem.ncbi.nlm.nih.gov | |
Description | Data deposited in or computed by PubChem | |
Isomeric SMILES |
C1=C(NC(=O)N(C1=O)[C@H]2[C@@H]([C@@H]([C@H](O2)CO)O)O)N | |
Source | PubChem | |
URL | https://pubchem.ncbi.nlm.nih.gov | |
Description | Data deposited in or computed by PubChem | |
Molecular Formula |
C9H13N3O6 | |
Source | PubChem | |
URL | https://pubchem.ncbi.nlm.nih.gov | |
Description | Data deposited in or computed by PubChem | |
DSSTOX Substance ID |
DTXSID80933948 | |
Record name | 6-Amino-2-hydroxy-3-pentofuranosylpyrimidin-4(3H)-one | |
Source | EPA DSSTox | |
URL | https://comptox.epa.gov/dashboard/DTXSID80933948 | |
Description | DSSTox provides a high quality public chemistry resource for supporting improved predictive toxicology. | |
Molecular Weight |
259.22 g/mol | |
Source | PubChem | |
URL | https://pubchem.ncbi.nlm.nih.gov | |
Description | Data deposited in or computed by PubChem | |
CAS No. |
150439-90-0 | |
Record name | 6-Oxocytidine | |
Source | ChemIDplus | |
URL | https://pubchem.ncbi.nlm.nih.gov/substance/?source=chemidplus&sourceid=0150439900 | |
Description | ChemIDplus is a free, web search system that provides access to the structure and nomenclature authority files used for the identification of chemical substances cited in National Library of Medicine (NLM) databases, including the TOXNET system. | |
Record name | 6-Amino-2-hydroxy-3-pentofuranosylpyrimidin-4(3H)-one | |
Source | EPA DSSTox | |
URL | https://comptox.epa.gov/dashboard/DTXSID80933948 | |
Description | DSSTox provides a high quality public chemistry resource for supporting improved predictive toxicology. | |
Disclaimer and Information on In-Vitro Research Products
Please be aware that all articles and product information presented on BenchChem are intended solely for informational purposes. The products available for purchase on BenchChem are specifically designed for in-vitro studies, which are conducted outside of living organisms. In-vitro studies, derived from the Latin term "in glass," involve experiments performed in controlled laboratory settings using cells or tissues. It is important to note that these products are not categorized as medicines or drugs, and they have not received approval from the FDA for the prevention, treatment, or cure of any medical condition, ailment, or disease. We must emphasize that any form of bodily introduction of these products into humans or animals is strictly prohibited by law. It is essential to adhere to these guidelines to ensure compliance with legal and ethical standards in research and experimentation.