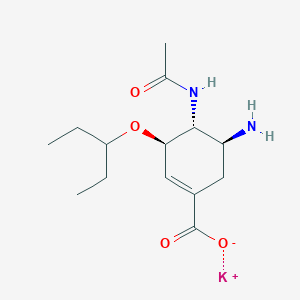
Oseltamivir Acid Potassium Salt
- Click on QUICK INQUIRY to receive a quote from our team of experts.
- With the quality product at a COMPETITIVE price, you can focus more on your research.
Overview
Description
Oseltamivir Acid Potassium Salt is a derivative of Oseltamivir, an antiviral medication widely known for its efficacy in treating and preventing influenza A and B. Oseltamivir works by inhibiting the neuraminidase enzyme, which is essential for the replication and spread of the influenza virus . The potassium salt form of Oseltamivir Acid is designed to enhance its solubility and stability, making it more effective for pharmaceutical applications.
Preparation Methods
Synthetic Routes and Reaction Conditions: The synthesis of Oseltamivir Acid Potassium Salt typically involves the following steps:
Formation of Oseltamivir Base: Oseltamivir base is synthesized through a series of chemical reactions starting from shikimic acid. This involves multiple steps including protection, oxidation, reduction, and cyclization reactions.
Conversion to Oseltamivir Acid: The Oseltamivir base is then hydrolyzed to form Oseltamivir Acid.
Salt Formation: The Oseltamivir Acid is treated with potassium hydroxide to form this compound.
Industrial Production Methods: Industrial production of this compound follows a similar synthetic route but is optimized for large-scale manufacturing. This includes:
Optimization of Reaction Conditions: Reaction conditions such as temperature, solvent, and reaction time are optimized to maximize yield and minimize impurities.
Purification: The product is purified through crystallization and filtration techniques to achieve pharmaceutical-grade purity.
Quality Control: Rigorous quality control measures are implemented to ensure the consistency and safety of the final product.
Chemical Reactions Analysis
Types of Reactions: Oseltamivir Acid Potassium Salt undergoes various chemical reactions, including:
Hydrolysis: Conversion of Oseltamivir base to Oseltamivir Acid.
Salt Formation: Reaction with potassium hydroxide to form the potassium salt.
Oxidation and Reduction: Involved in the initial synthesis of Oseltamivir base from shikimic acid.
Common Reagents and Conditions:
Hydrolysis: Typically carried out in aqueous or organic solvents under acidic or basic conditions.
Salt Formation: Involves the use of potassium hydroxide in an organic solvent like ethyl acetate.
Oxidation and Reduction: Various oxidizing and reducing agents are used in the multi-step synthesis of Oseltamivir base.
Major Products Formed:
Oseltamivir Acid: Formed through hydrolysis of Oseltamivir base.
This compound: Formed through the reaction of Oseltamivir Acid with potassium hydroxide.
Scientific Research Applications
Oseltamivir Acid Potassium Salt has a wide range of applications in scientific research, including:
Chemistry: Used as a model compound for studying antiviral drug synthesis and mechanisms.
Biology: Investigated for its effects on viral replication and interaction with cellular components.
Medicine: Extensively used in the development of antiviral therapies for influenza and other viral infections.
Industry: Employed in the formulation of pharmaceutical products for the treatment and prevention of influenza.
Mechanism of Action
Oseltamivir Acid Potassium Salt exerts its antiviral effects by inhibiting the neuraminidase enzyme on the surface of the influenza virus. This enzyme is crucial for the release of new viral particles from infected cells. By blocking neuraminidase activity, Oseltamivir prevents the spread of the virus within the host, thereby reducing the severity and duration of the infection .
Comparison with Similar Compounds
Zanamivir: Another neuraminidase inhibitor used to treat influenza.
Peramivir: An intravenous neuraminidase inhibitor for severe influenza cases.
Laninamivir: A long-acting neuraminidase inhibitor used for influenza prophylaxis.
Uniqueness of Oseltamivir Acid Potassium Salt:
Oral Bioavailability: Unlike Zanamivir, which is inhaled, Oseltamivir can be administered orally, making it more convenient for patients.
Stability and Solubility: The potassium salt form enhances the stability and solubility of Oseltamivir Acid, improving its efficacy and shelf-life.
Biological Activity
Oseltamivir Acid Potassium Salt, commonly known as oseltamivir phosphate, is an antiviral medication primarily used for the treatment and prevention of influenza. This compound acts by inhibiting the neuraminidase enzyme, which is crucial for the viral replication cycle. The biological activity of oseltamivir involves several pharmacological and biochemical mechanisms that are critical for its therapeutic efficacy.
Oseltamivir is a prodrug that is rapidly converted in the liver to its active form, oseltamivir carboxylate (OC). This active metabolite selectively inhibits the neuraminidase enzymes on the surface of influenza viruses, preventing the release of new virions from infected cells. By blocking this enzyme, oseltamivir effectively reduces viral spread within the respiratory tract, thereby alleviating symptoms and shortening the duration of illness.
Pharmacokinetics
The pharmacokinetic profile of oseltamivir reveals important insights into its absorption, distribution, metabolism, and excretion:
- Absorption : Oseltamivir is well absorbed from the gastrointestinal tract with an absolute bioavailability of approximately 80% after oral administration .
- Metabolism : It is converted to OC predominantly by hepatic esterases. OC is the primary active metabolite and is responsible for the antiviral effects .
- Distribution : The volume of distribution for OC ranges from 23 to 26 liters, indicating extensive distribution in body tissues .
- Elimination : Oseltamivir and OC are primarily eliminated via renal excretion. The elimination half-life for oseltamivir is about 1–3 hours, while OC persists longer with a half-life of 6–10 hours .
Inhibition of Neuraminidase
Oseltamivir's primary biological activity is its ability to inhibit viral neuraminidase. Studies have shown that it effectively blocks this enzyme at low nanomolar concentrations, significantly more potent than its effects on human sialidases . This selectivity is crucial as it minimizes potential side effects related to human enzyme inhibition.
Other Biological Activities
Recent studies have explored additional biological activities of oseltamivir and its derivatives:
- Acetylcholinesterase Inhibition : Oseltamivir has shown some inhibitory activity against acetylcholinesterase, which may have implications for neurodegenerative diseases like Alzheimer's .
- Cytotoxicity : Research indicates that certain derivatives of oseltamivir exhibit cytotoxic effects against specific cancer cell lines, suggesting potential applications beyond antiviral therapy .
Case Studies
A review of clinical data highlights the effectiveness and safety profile of oseltamivir in treating influenza:
- Case Study 1 : A cohort study involving patients treated with oseltamivir showed a significant reduction in hospitalization rates due to influenza-related complications.
- Case Study 2 : In a randomized controlled trial, patients receiving oseltamivir reported a shorter duration of symptoms compared to those receiving placebo, reinforcing its efficacy in acute influenza management.
Properties
IUPAC Name |
potassium;(3R,4R,5S)-4-acetamido-5-amino-3-pentan-3-yloxycyclohexene-1-carboxylate |
Source
|
---|---|---|
Source | PubChem | |
URL | https://pubchem.ncbi.nlm.nih.gov | |
Description | Data deposited in or computed by PubChem | |
InChI |
InChI=1S/C14H24N2O4.K/c1-4-10(5-2)20-12-7-9(14(18)19)6-11(15)13(12)16-8(3)17;/h7,10-13H,4-6,15H2,1-3H3,(H,16,17)(H,18,19);/q;+1/p-1/t11-,12+,13+;/m0./s1 |
Source
|
Source | PubChem | |
URL | https://pubchem.ncbi.nlm.nih.gov | |
Description | Data deposited in or computed by PubChem | |
InChI Key |
MGDJOHGESXQWMU-LUHWTZLKSA-M |
Source
|
Source | PubChem | |
URL | https://pubchem.ncbi.nlm.nih.gov | |
Description | Data deposited in or computed by PubChem | |
Canonical SMILES |
CCC(CC)OC1C=C(CC(C1NC(=O)C)N)C(=O)[O-].[K+] |
Source
|
Source | PubChem | |
URL | https://pubchem.ncbi.nlm.nih.gov | |
Description | Data deposited in or computed by PubChem | |
Isomeric SMILES |
CCC(CC)O[C@@H]1C=C(C[C@@H]([C@H]1NC(=O)C)N)C(=O)[O-].[K+] |
Source
|
Source | PubChem | |
URL | https://pubchem.ncbi.nlm.nih.gov | |
Description | Data deposited in or computed by PubChem | |
Molecular Formula |
C14H23KN2O4 |
Source
|
Source | PubChem | |
URL | https://pubchem.ncbi.nlm.nih.gov | |
Description | Data deposited in or computed by PubChem | |
Molecular Weight |
322.44 g/mol |
Source
|
Source | PubChem | |
URL | https://pubchem.ncbi.nlm.nih.gov | |
Description | Data deposited in or computed by PubChem | |
Disclaimer and Information on In-Vitro Research Products
Please be aware that all articles and product information presented on BenchChem are intended solely for informational purposes. The products available for purchase on BenchChem are specifically designed for in-vitro studies, which are conducted outside of living organisms. In-vitro studies, derived from the Latin term "in glass," involve experiments performed in controlled laboratory settings using cells or tissues. It is important to note that these products are not categorized as medicines or drugs, and they have not received approval from the FDA for the prevention, treatment, or cure of any medical condition, ailment, or disease. We must emphasize that any form of bodily introduction of these products into humans or animals is strictly prohibited by law. It is essential to adhere to these guidelines to ensure compliance with legal and ethical standards in research and experimentation.