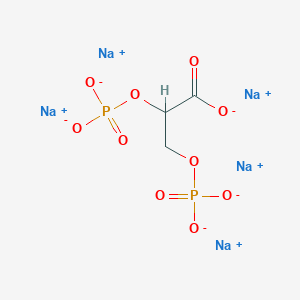
2,3-Diphospho-Glyceric Acid Pentasodium
- Click on QUICK INQUIRY to receive a quote from our team of experts.
- With the quality product at a COMPETITIVE price, you can focus more on your research.
Overview
Description
2,3-Diphospho-D-glyceric acid pentasodium salt (2,3-DPG pentasodium) is a biologically significant compound with the molecular formula C₃H₃Na₅O₁₀P₂ and a molecular weight of 375.95 g/mol (CAS: 102783-53-9) . It is a phosphorylated derivative of glyceric acid, featuring two phosphate groups at the 2- and 3-positions of the glycerol backbone. This compound plays a critical role in regulating oxygen transport in red blood cells by modulating hemoglobin’s affinity for oxygen .
In laboratory settings, 2,3-DPG pentasodium is utilized as:
Preparation Methods
Synthetic Routes and Reaction Conditions: The synthesis of 2,3-Diphospho-Glyceric Acid Pentasodium involves the phosphorylation of glyceric acid. The reaction typically requires phosphorylating agents such as phosphoric acid or its derivatives under controlled conditions to ensure the formation of the diphosphate ester. The reaction is carried out in an aqueous medium, and the product is purified through crystallization or other suitable methods .
Industrial Production Methods: Industrial production of this compound follows similar synthetic routes but on a larger scale. The process involves the use of high-purity reagents and stringent quality control measures to ensure the consistency and purity of the final product. The compound is typically produced in powder form and stored at low temperatures to maintain its stability .
Chemical Reactions Analysis
Types of Reactions: 2,3-Diphospho-Glyceric Acid Pentasodium undergoes various chemical reactions, including:
Oxidation: The compound can be oxidized to form corresponding phosphoric acid derivatives.
Reduction: Reduction reactions can convert the diphosphate groups to monophosphate or free hydroxyl groups.
Substitution: The diphosphate groups can be substituted with other functional groups under specific conditions.
Common Reagents and Conditions:
Oxidation: Common oxidizing agents include potassium permanganate and hydrogen peroxide.
Reduction: Reducing agents such as sodium borohydride or lithium aluminum hydride are used.
Substitution: Substitution reactions often require catalysts or specific pH conditions to proceed efficiently.
Major Products: The major products formed from these reactions include various phosphoric acid derivatives, monophosphate esters, and substituted glyceric acid compounds .
Scientific Research Applications
2,3-Diphospho-Glyceric Acid Pentasodium has a wide range of applications in scientific research:
Chemistry: It is used as a reference compound in the analysis of blood cell glycolytic cycle metabolites.
Biology: The compound is crucial in studying erythrocyte metabolism and hemoglobin function.
Medicine: It is used in research related to blood disorders and oxygen transport mechanisms.
Industry: The compound finds applications in the production of biochemical reagents and diagnostic kits.
Mechanism of Action
2,3-Diphospho-Glyceric Acid Pentasodium exerts its effects by binding to hemoglobin and reducing its affinity for oxygen. This binding stabilizes the deoxygenated form of hemoglobin, facilitating the release of oxygen at tissue sites. The compound acts as an allosteric regulator, influencing the oxygen-binding capacity of hemoglobin and thus playing a vital role in oxygen transport and delivery .
Comparison with Similar Compounds
The following section compares 2,3-DPG pentasodium with structurally or functionally related pentasodium salts, emphasizing differences in applications, chemical properties, and regulatory roles.
Diethylenetriamine Pentaacetic Acid Pentasodium Salt (DTPA)
Key Differences :
DTPA pentasodium lacks the phosphate groups critical to 2,3-DPG’s role in oxygen transport. Instead, its five carboxylate groups enable strong metal chelation, making it suitable for industrial and analytical applications (e.g., stabilizing lab reagents) .
Pentasodium Triphosphate
Listed in pharmacopeial documents (), pentasodium triphosphate (Na₅P₃O₁₀) is an inorganic salt with distinct industrial applications:
- Function : Water softening, detergent formulation.
- Key Difference : Unlike 2,3-DPG, it lacks organic backbone and biochemical relevance, focusing instead on phosphate-based sequestration .
Pentetate Pentasodium
Another pharmacopeial compound (), pentetate pentasodium shares DTPA’s chelation properties but differs structurally. Limited data in the provided evidence precludes direct comparison with 2,3-DPG .
Research Implications
- 2,3-DPG’s unique phosphorylated structure underpins its role in metabolic pathways, distinguishing it from chelators like DTPA or industrial salts like pentasodium triphosphate.
Biological Activity
2,3-Diphospho-glyceric acid pentasodium (2,3-DPG) is a crucial metabolite in human physiology, primarily known for its role in regulating hemoglobin's oxygen affinity. This compound is a sodium salt of 2,3-diphosphoglyceric acid, which is involved in various biological processes, particularly in red blood cells (RBCs). This article explores the biological activity of 2,3-DPG, its enzymatic interactions, physiological effects, and potential therapeutic applications.
Overview of this compound
2,3-DPG is synthesized from 1,3-bisphosphoglycerate through the action of bisphosphoglycerate mutase and plays a vital role in the glycolytic pathway. It exists at high concentrations in erythrocytes and functions as an allosteric effector that modulates hemoglobin's oxygen-binding properties. This compound facilitates oxygen release from hemoglobin to tissues that require it most, particularly under conditions of hypoxia or increased metabolic demand .
Enzymatic Interactions
The biological activity of 2,3-DPG is mediated by several enzymes:
- Bisphosphoglycerate Mutase (BPGM) : Converts 1,3-bisphosphoglycerate to 2,3-DPG. It plays a critical role in regulating the levels of 2,3-DPG in RBCs.
- Bisphosphoglycerate Phosphatase : Catalyzes the dephosphorylation of 2,3-DPG to produce 3-phosphoglycerate. This reaction is essential for maintaining the balance of glycolytic intermediates and energy production .
Table: Enzymatic Reactions Involving 2,3-DPG
Enzyme | Reaction Description | Gene Name | Uniprot ID |
---|---|---|---|
Bisphosphoglycerate Mutase | Converts 1,3-BPG to 2,3-DPG | BPGM | P07738 |
Bisphosphoglycerate Phosphatase | Converts 2,3-DPG to 3-PG | MINPP1 | Q9UNW1 |
Physiological Effects
The primary physiological effect of 2,3-DPG is its ability to decrease hemoglobin's affinity for oxygen. This mechanism is crucial during various physiological states:
- Hypoxia : In conditions where oxygen levels are low (e.g., chronic respiratory diseases), RBCs increase their intracellular concentration of 2,3-DPG to enhance oxygen delivery to tissues .
- High Altitude : At high altitudes where oxygen availability is reduced, increased levels of 2,3-DPG facilitate greater oxygen release from hemoglobin .
- Fetal-Maternal Oxygen Transfer : Fetal blood has lower levels of 2,3-DPG compared to maternal blood. This difference enhances oxygen uptake by fetal blood in the placenta .
Case Studies and Research Findings
- Regulation of Oxygen Affinity : A study demonstrated that modulation of phosphatase/synthase activities could directly regulate the levels of 2,3-DPG in erythrocytes. Increased levels were associated with a rightward shift in the oxygen-hemoglobin dissociation curve .
- Impact on Malaria Parasites : Research showed that synthetic forms of 2,3-DPG inhibited the growth of Plasmodium falciparum, suggesting potential applications in treating malaria by disrupting parasite metabolism without affecting host cells .
Q & A
Basic Research Questions
Q. What are the optimal storage and preparation protocols for 2,3-Diphospho-Glyceric Acid Pentasodium to ensure stability in experimental settings?
- Methodological Answer : The compound should be stored as a powder at -20°C for long-term stability (up to 3 years) or at 4°C for shorter durations (up to 2 years). For solubility, prepare stock solutions in DMSO, water, ethanol, or DMF, depending on the experimental system. In vivo formulations, such as injectable solutions, require sterile PBS (pH 7.4) and brief vortexing followed by ultrasonication to ensure homogeneity. Avoid repeated freeze-thaw cycles in solvent-based stocks; store working aliquots at -80°C for up to 6 months .
Q. How does this compound modulate hemoglobin-oxygen affinity, and what experimental techniques validate this interaction?
- Methodological Answer : The compound binds to hemoglobin’s central cavity, reducing oxygen affinity by stabilizing the deoxygenated T-state. This mechanism is validated using oxygen dissociation curves measured via tonometry or spectrophotometry. For precise quantification, combine hemoglobin purification (e.g., via size-exclusion chromatography) with isothermal titration calorimetry (ITC) to assess binding constants. Control experiments should include parallel assays with erythrocyte lysates to account for endogenous 2,3-DPG interference .
Q. What are the key considerations for integrating 2,3-DPG Pentasodium into enzymatic assays, such as glycolysis studies?
- Methodological Answer : Use the compound as a substrate or modulator in assays targeting phosphoglycerate mutase or bisphosphoglycerate phosphatase. Prepare reaction buffers (e.g., Tris-HCl, pH 7.4) with 1–5 mM MgCl₂ to stabilize enzyme activity. Monitor reaction progress via coupled NADH/NAD+ absorbance at 340 nm or HPLC-based phosphate quantification. Include negative controls with 3-phosphoglyceric acid (a common impurity) to rule out off-target effects .
Advanced Research Questions
Q. How can researchers resolve contradictions in reported effects of 2,3-DPG Pentasodium on vascular calcification (VC) across different cell models?
- Methodological Answer : Discrepancies may arise from variations in cell type (e.g., primary VSMCs vs. immortalized MOVAS lines) or calcification induction methods (e.g., high-phosphate media vs. β-glycerophosphate). Standardize protocols by:
- Pre-treating cells with 2,3-DPG (10–100 µM) for 24 hours before calcification induction.
- Quantifying calcium deposition via Alizarin Red staining and atomic absorption spectroscopy.
- Validating results with orthogonal techniques, such as alkaline phosphatase activity assays or qPCR for osteogenic markers (e.g., RUNX2). Cross-reference outcomes with in vivo models (e.g., CKD rodent models) to confirm physiological relevance .
Q. What experimental frameworks (e.g., PICO, FINER) are recommended for designing studies on 2,3-DPG Pentasodium’s role in metabolic reprogramming?
- Methodological Answer : Apply the PICO framework to structure hypotheses:
- Population : Cell lines (e.g., cancer or erythroid progenitors).
- Intervention : 2,3-DPG supplementation (dose range: 0.1–10 mM).
- Comparison : Untreated controls or 3-phosphoglycerate-treated groups.
- Outcome : Glycolytic flux (measured via Seahorse XF Analyzer) or 2,3-DPG levels (via LC-MS/MS).
Use the FINER criteria to ensure feasibility (e.g., cost of isotopic labeling for tracer studies) and novelty (e.g., exploring 2,3-DPG’s impact on HIF-1α stabilization under hypoxia) .
Q. What advanced techniques are critical for elucidating 2,3-DPG Pentasodium’s structural interactions with biomolecules?
- Methodological Answer : Employ X-ray crystallography or cryo-EM to resolve binding sites on hemoglobin or enzymes. For dynamic studies, use molecular dynamics simulations (e.g., GROMACS) to model conformational changes upon 2,3-DPG binding. Pair these with surface plasmon resonance (SPR) to quantify kinetic parameters (ka, kd). Validate findings using mutagenesis (e.g., Hb mutants lacking 2,3-DPG binding residues) .
Q. Methodological Notes
- Interference Mitigation : In assays with serum-containing media, pre-treat samples with phosphatases (e.g., calf intestinal phosphatase) to degrade endogenous phosphorylated metabolites that may confound results .
- Data Reproducibility : Report batch-specific purity levels (e.g., ≥95% via HPLC) and impurity profiles (e.g., 3-phosphoglyceric acid content) to enhance cross-study comparability .
Properties
IUPAC Name |
pentasodium;2,3-diphosphonatooxypropanoate |
Source
|
---|---|---|
Source | PubChem | |
URL | https://pubchem.ncbi.nlm.nih.gov | |
Description | Data deposited in or computed by PubChem | |
InChI |
InChI=1S/C3H8O10P2.5Na/c4-3(5)2(13-15(9,10)11)1-12-14(6,7)8;;;;;/h2H,1H2,(H,4,5)(H2,6,7,8)(H2,9,10,11);;;;;/q;5*+1/p-5 |
Source
|
Source | PubChem | |
URL | https://pubchem.ncbi.nlm.nih.gov | |
Description | Data deposited in or computed by PubChem | |
InChI Key |
KPRMRMXBVAUXKZ-UHFFFAOYSA-I |
Source
|
Source | PubChem | |
URL | https://pubchem.ncbi.nlm.nih.gov | |
Description | Data deposited in or computed by PubChem | |
Canonical SMILES |
C(C(C(=O)[O-])OP(=O)([O-])[O-])OP(=O)([O-])[O-].[Na+].[Na+].[Na+].[Na+].[Na+] |
Source
|
Source | PubChem | |
URL | https://pubchem.ncbi.nlm.nih.gov | |
Description | Data deposited in or computed by PubChem | |
Molecular Formula |
C3H3Na5O10P2 |
Source
|
Source | PubChem | |
URL | https://pubchem.ncbi.nlm.nih.gov | |
Description | Data deposited in or computed by PubChem | |
Molecular Weight |
375.95 g/mol |
Source
|
Source | PubChem | |
URL | https://pubchem.ncbi.nlm.nih.gov | |
Description | Data deposited in or computed by PubChem | |
Disclaimer and Information on In-Vitro Research Products
Please be aware that all articles and product information presented on BenchChem are intended solely for informational purposes. The products available for purchase on BenchChem are specifically designed for in-vitro studies, which are conducted outside of living organisms. In-vitro studies, derived from the Latin term "in glass," involve experiments performed in controlled laboratory settings using cells or tissues. It is important to note that these products are not categorized as medicines or drugs, and they have not received approval from the FDA for the prevention, treatment, or cure of any medical condition, ailment, or disease. We must emphasize that any form of bodily introduction of these products into humans or animals is strictly prohibited by law. It is essential to adhere to these guidelines to ensure compliance with legal and ethical standards in research and experimentation.