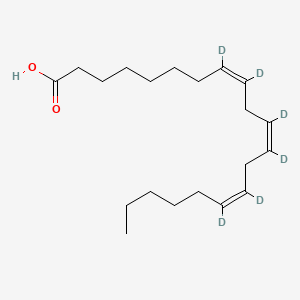
Dihomo-|A-linolenic Acid-d6
- Click on QUICK INQUIRY to receive a quote from our team of experts.
- With the quality product at a COMPETITIVE price, you can focus more on your research.
Overview
Description
Dihomo-γ-Linolenic Acid-d6 is a deuterated form of Dihomo-γ-Linolenic Acid, a polyunsaturated fatty acid with 20 carbon atoms and three cis double bonds. This compound is significant in the study of metabolic pathways and inflammation due to its role as a precursor to various bioactive lipids .
Mechanism of Action
Target of Action
Dihomo-γ-linolenic Acid-d6 (DGLA-d6) primarily targets the cyclooxygenase pathway . It is a significant molecule differentiating healthy and inflamed tissues .
Mode of Action
DGLA-d6 interacts with its targets by being converted into prostaglandin E1 (PGE1) through the cyclooxygenase pathway . PGE1 inhibits platelet aggregation and also exerts a vasodilatory effect .
Biochemical Pathways
DGLA-d6 is a pivotal point of metabolic pathways leading to anti-inflammatory derivatives or via arachidonic acid (ARA) to pro-inflammatory lipid mediators . The balance of ARA to DGLA is probably a critical factor affecting inflammatory processes in the body .
Pharmacokinetics
It is known that dgla can be produced by cultured fungi or be obtained via endogenous conversion from γ-linolenic acid (gla)-rich vegetable oils .
Result of Action
The result of DGLA-d6’s action is the production of PGE1, which inhibits platelet aggregation and exerts a vasodilatory effect . This leads to anti-inflammatory and anti-proliferative activities .
Action Environment
The action of DGLA-d6 can be influenced by various factors. For instance, several disease states are characterized by abnormally low DGLA levels in the body, while others can feature elevated levels . A defect in the activity of ∆6-desaturase and/or ∆5-desaturase may be one factor in the initiation and progression of these conditions .
Biochemical Analysis
Biochemical Properties
Dihomo-γ-linolenic Acid-d6 plays a crucial role in biochemical reactions. It is a substrate for the enzymes cyclooxygenase and lipoxygenase . The interactions between Dihomo-γ-linolenic Acid-d6 and these enzymes lead to the production of various eicosanoids, which are signaling molecules that have wide-ranging effects on the body’s cardiovascular, immune, and inflammatory responses .
Cellular Effects
Dihomo-γ-linolenic Acid-d6 has significant effects on various types of cells and cellular processes. It influences cell function by affecting cell signaling pathways, gene expression, and cellular metabolism . For instance, it has anti-inflammatory and anti-proliferative activities, and it can attenuate atherosclerosis in the apolipoprotein E deficient mouse model system .
Molecular Mechanism
The molecular mechanism of Dihomo-γ-linolenic Acid-d6 involves its conversion into prostaglandin E1 (PGE1) through the cyclooxygenase pathway . PGE1 inhibits platelet aggregation and also exerts a vasodilatory effect . This mechanism of action allows Dihomo-γ-linolenic Acid-d6 to exert its effects at the molecular level, including binding interactions with biomolecules, enzyme inhibition or activation, and changes in gene expression .
Metabolic Pathways
Dihomo-γ-linolenic Acid-d6 is involved in several metabolic pathways. It can be converted to arachidonic acid (AA), another 20-carbon ω-6 PUFA . This conversion involves various enzymes and cofactors . The balance of AA to DGLA is a critical factor affecting inflammatory processes in the body .
Preparation Methods
Synthetic Routes and Reaction Conditions
Dihomo-γ-Linolenic Acid-d6 can be synthesized by the elongation of γ-Linolenic Acid, which involves the addition of two carbon atoms to the γ-Linolenic Acid molecule. This process is typically catalyzed by fatty acid elongases . The deuterated form, Dihomo-γ-Linolenic Acid-d6, is prepared by incorporating deuterium atoms at specific positions in the molecule. This is achieved through the use of deuterated reagents and solvents during the synthesis .
Industrial Production Methods
Industrial production of Dihomo-γ-Linolenic Acid-d6 involves the use of biotechnological methods, such as the fermentation of genetically modified microorganisms that can produce the desired fatty acid. These microorganisms are cultured in deuterated media to incorporate deuterium into the fatty acid .
Chemical Reactions Analysis
Types of Reactions
Dihomo-γ-Linolenic Acid-d6 undergoes various chemical reactions, including oxidation, reduction, and substitution .
Common Reagents and Conditions
Oxidation: Common oxidizing agents include oxygen and peroxides.
Substitution: Halogenation reactions can be carried out using halogens like chlorine or bromine under controlled conditions.
Major Products Formed
The major products formed from these reactions include various oxygenated derivatives, reduced forms of the fatty acid, and halogenated compounds .
Scientific Research Applications
Dihomo-γ-Linolenic Acid-d6 is used extensively in scientific research due to its role in metabolic pathways and its anti-inflammatory properties .
Chemistry
In chemistry, it is used as a standard in mass spectrometry for the quantification of Dihomo-γ-Linolenic Acid .
Biology
In biological research, it is used to study the metabolism of polyunsaturated fatty acids and their role in cellular processes .
Medicine
In medicine, it is investigated for its potential therapeutic effects in inflammatory diseases, such as rheumatoid arthritis and atopic dermatitis .
Industry
In the industry, it is used in the production of specialized dietary supplements and pharmaceuticals .
Comparison with Similar Compounds
Similar Compounds
Arachidonic Acid: Another polyunsaturated fatty acid with 20 carbon atoms, but with four cis double bonds.
γ-Linolenic Acid: An 18-carbon fatty acid with three cis double bonds.
Linoleic Acid: An 18-carbon fatty acid with two cis double bonds.
Uniqueness
Dihomo-γ-Linolenic Acid-d6 is unique due to its deuterated form, which makes it a valuable tool in mass spectrometry and metabolic studies. Its role as a precursor to both anti-inflammatory and pro-inflammatory mediators also distinguishes it from other similar fatty acids .
Properties
IUPAC Name |
(8Z,11Z,14Z)-8,9,11,12,14,15-hexadeuterioicosa-8,11,14-trienoic acid |
Source
|
---|---|---|
Source | PubChem | |
URL | https://pubchem.ncbi.nlm.nih.gov | |
Description | Data deposited in or computed by PubChem | |
InChI |
InChI=1S/C20H34O2/c1-2-3-4-5-6-7-8-9-10-11-12-13-14-15-16-17-18-19-20(21)22/h6-7,9-10,12-13H,2-5,8,11,14-19H2,1H3,(H,21,22)/b7-6-,10-9-,13-12-/i6D,7D,9D,10D,12D,13D |
Source
|
Source | PubChem | |
URL | https://pubchem.ncbi.nlm.nih.gov | |
Description | Data deposited in or computed by PubChem | |
InChI Key |
HOBAELRKJCKHQD-JALKMEHMSA-N |
Source
|
Source | PubChem | |
URL | https://pubchem.ncbi.nlm.nih.gov | |
Description | Data deposited in or computed by PubChem | |
Canonical SMILES |
CCCCCC=CCC=CCC=CCCCCCCC(=O)O |
Source
|
Source | PubChem | |
URL | https://pubchem.ncbi.nlm.nih.gov | |
Description | Data deposited in or computed by PubChem | |
Isomeric SMILES |
[2H]/C(=C(\[2H])/C/C(=C(/[2H])\C/C(=C(/[2H])\CCCCCCC(=O)O)/[2H])/[2H])/CCCCC |
Source
|
Source | PubChem | |
URL | https://pubchem.ncbi.nlm.nih.gov | |
Description | Data deposited in or computed by PubChem | |
Molecular Formula |
C20H34O2 |
Source
|
Source | PubChem | |
URL | https://pubchem.ncbi.nlm.nih.gov | |
Description | Data deposited in or computed by PubChem | |
Molecular Weight |
312.5 g/mol |
Source
|
Source | PubChem | |
URL | https://pubchem.ncbi.nlm.nih.gov | |
Description | Data deposited in or computed by PubChem | |
Q1: Why is Dihomo-γ-linolenic Acid-d6 used in the research instead of the non-deuterated Dihomo-γ-linolenic Acid?
A1: The research utilizes Dihomo-γ-linolenic Acid-d6 as an internal standard for the quantification of Dihomo-γ-linolenic Acid in serum samples using LC-MS/MS. [] Internal standards are crucial in mass spectrometry to correct for variations during sample preparation and ionization. Deuterated analogs, like Dihomo-γ-linolenic Acid-d6, are chemically similar to the target analyte but have a different mass due to the presence of heavier deuterium atoms. This mass difference allows for differentiation during mass spectrometry analysis, while the similar chemical behavior ensures that the internal standard undergoes similar extraction and ionization efficiencies as the target analyte.
Q2: What analytical method is used to detect Dihomo-γ-linolenic Acid and its deuterated counterpart in the study?
A2: The research employs Liquid Chromatography coupled with tandem Mass Spectrometry (LC-MS/MS) to detect and quantify Dihomo-γ-linolenic Acid and Dihomo-γ-linolenic Acid-d6. [] This technique combines the separation capabilities of liquid chromatography with the sensitivity and selectivity of mass spectrometry.
Disclaimer and Information on In-Vitro Research Products
Please be aware that all articles and product information presented on BenchChem are intended solely for informational purposes. The products available for purchase on BenchChem are specifically designed for in-vitro studies, which are conducted outside of living organisms. In-vitro studies, derived from the Latin term "in glass," involve experiments performed in controlled laboratory settings using cells or tissues. It is important to note that these products are not categorized as medicines or drugs, and they have not received approval from the FDA for the prevention, treatment, or cure of any medical condition, ailment, or disease. We must emphasize that any form of bodily introduction of these products into humans or animals is strictly prohibited by law. It is essential to adhere to these guidelines to ensure compliance with legal and ethical standards in research and experimentation.