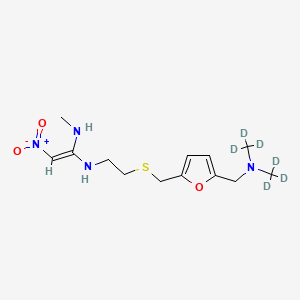
Ranitidine-d6
- Click on QUICK INQUIRY to receive a quote from our team of experts.
- With the quality product at a COMPETITIVE price, you can focus more on your research.
Overview
Description
Ranitidine-d6 is a deuterated form of ranitidine, a histamine H2 receptor antagonist. It is primarily used as an internal standard in the quantification of ranitidine by gas chromatography (GC) or liquid chromatography-mass spectrometry (LC-MS). Ranitidine itself is widely known for its use in treating conditions such as gastroesophageal reflux disease (GERD), peptic ulcers, and Zollinger-Ellison syndrome .
Mechanism of Action
Target of Action
Ranitidine-d6 primarily targets the histamine H2 receptors found on gastric parietal cells . These receptors play a crucial role in the secretion of gastric acid, which is essential for digestion .
Mode of Action
This compound interacts with its targets by reversibly binding to the histamine H2 receptors . This binding inhibits the action of histamine, leading to a reduction in gastric acid secretion . This interaction results in a decrease in the volume and concentration of gastric acid, thereby reducing the symptoms associated with conditions like gastroesophageal reflux disease (GERD) and peptic ulcers .
Biochemical Pathways
This compound affects the biochemical pathway involving histamine and its action on the H2 receptors . By blocking these receptors, this compound inhibits the normal pathway of gastric acid secretion stimulated by histamine . This leads to a decrease in the production of gastric acid and pepsin, a digestive enzyme .
Pharmacokinetics
The pharmacokinetics of this compound involves its absorption, distribution, metabolism, and excretion (ADME). After oral administration, about 50% of this compound is absorbed . It is distributed throughout the body but minimally penetrates the blood-brain barrier . This compound undergoes minor metabolism in the liver to form N-oxide, S-oxide, and N-desmethyl metabolites . About 30% of the drug is excreted unchanged in the urine .
Result of Action
The molecular and cellular effects of this compound’s action primarily involve the reduction of gastric acid secretion . This leads to an improvement in the symptoms of conditions associated with excess gastric acid, such as heartburn and acid indigestion .
Action Environment
Environmental factors can influence the action, efficacy, and stability of this compound. For instance, the presence of nitrate ions, the acidity of the environment, and nitrate contamination of pharmaceutical excipients can affect the production of N-nitrosodimethylamine, a probable human carcinogen, in this compound . Temperature is another factor that can influence the stability of this compound . Therefore, these factors should be carefully controlled during the manufacturing and storage of this compound to ensure its safety and efficacy.
Biochemical Analysis
Biochemical Properties
Ranitidine-d6, like its non-deuterated counterpart, interacts with histamine H2 receptors . It reverses histamine-induced relaxation of isolated rat uterine horn and histamine-induced increases in contraction frequency in isolated guinea pig right atrium .
Cellular Effects
This compound, by virtue of its antagonistic action on histamine H2 receptors, influences cell function by modulating the effects of histamine, a key signaling molecule involved in numerous cellular processes .
Molecular Mechanism
The molecular mechanism of action of this compound involves binding to histamine H2 receptors, thereby inhibiting the action of histamine . This results in changes in cell signaling and gene expression related to the effects of histamine .
Metabolic Pathways
This compound, like Ranitidine, is likely to be involved in metabolic pathways related to the metabolism of histamine, given its role as a histamine H2 receptor antagonist .
Preparation Methods
Synthetic Routes and Reaction Conditions
The synthesis of ranitidine-d6 involves the incorporation of deuterium atoms into the ranitidine molecule. This can be achieved through various methods, including the use of deuterated reagents or solvents during the synthesis process. One common approach is to use deuterated methylamine in the reaction with the appropriate intermediates to produce this compound .
Industrial Production Methods
Industrial production of this compound follows similar principles as laboratory synthesis but on a larger scale. The process involves the use of deuterated reagents and solvents in controlled reaction conditions to ensure the incorporation of deuterium atoms. The final product is then purified and characterized to confirm its isotopic purity and chemical structure .
Chemical Reactions Analysis
Types of Reactions
Ranitidine-d6, like its non-deuterated counterpart, can undergo various chemical reactions, including:
Oxidation: Ranitidine can be oxidized to form N-oxide derivatives.
Reduction: Reduction reactions can convert ranitidine to its corresponding amine derivatives.
Substitution: Substitution reactions can occur at the nitro group or the furan ring
Common Reagents and Conditions
Common reagents used in these reactions include oxidizing agents like hydrogen peroxide for oxidation, reducing agents like sodium borohydride for reduction, and nucleophiles for substitution reactions. The reaction conditions typically involve controlled temperatures and pH levels to ensure the desired transformation .
Major Products Formed
The major products formed from these reactions depend on the specific reaction conditions and reagents used. For example, oxidation of ranitidine can yield N-oxide derivatives, while reduction can produce amine derivatives .
Scientific Research Applications
Ranitidine-d6 is primarily used as an internal standard in analytical chemistry for the quantification of ranitidine in various samples. Its applications extend to:
Chemistry: Used in the development and validation of analytical methods for ranitidine quantification.
Biology: Employed in pharmacokinetic studies to monitor the concentration of ranitidine in biological samples.
Medicine: Utilized in clinical research to study the pharmacodynamics and pharmacokinetics of ranitidine.
Industry: Applied in quality control processes to ensure the accuracy and precision of ranitidine measurements in pharmaceutical formulations .
Comparison with Similar Compounds
Similar Compounds
Ranitidine-d6 can be compared with other histamine H2 receptor antagonists, such as:
- Cimetidine
- Famotidine
- Nizatidine
Uniqueness
The uniqueness of this compound lies in its deuterium labeling, which makes it an ideal internal standard for analytical methods. This isotopic labeling provides enhanced accuracy and precision in the quantification of ranitidine, making it a valuable tool in scientific research and industrial applications .
Properties
IUPAC Name |
(E)-1-N'-[2-[[5-[[bis(trideuteriomethyl)amino]methyl]furan-2-yl]methylsulfanyl]ethyl]-1-N-methyl-2-nitroethene-1,1-diamine |
Source
|
---|---|---|
Source | PubChem | |
URL | https://pubchem.ncbi.nlm.nih.gov | |
Description | Data deposited in or computed by PubChem | |
InChI |
InChI=1S/C13H22N4O3S/c1-14-13(9-17(18)19)15-6-7-21-10-12-5-4-11(20-12)8-16(2)3/h4-5,9,14-15H,6-8,10H2,1-3H3/b13-9+/i2D3,3D3 |
Source
|
Source | PubChem | |
URL | https://pubchem.ncbi.nlm.nih.gov | |
Description | Data deposited in or computed by PubChem | |
InChI Key |
VMXUWOKSQNHOCA-RUESZMOGSA-N |
Source
|
Source | PubChem | |
URL | https://pubchem.ncbi.nlm.nih.gov | |
Description | Data deposited in or computed by PubChem | |
Canonical SMILES |
CNC(=C[N+](=O)[O-])NCCSCC1=CC=C(O1)CN(C)C |
Source
|
Source | PubChem | |
URL | https://pubchem.ncbi.nlm.nih.gov | |
Description | Data deposited in or computed by PubChem | |
Isomeric SMILES |
[2H]C([2H])([2H])N(CC1=CC=C(O1)CSCCN/C(=C/[N+](=O)[O-])/NC)C([2H])([2H])[2H] |
Source
|
Source | PubChem | |
URL | https://pubchem.ncbi.nlm.nih.gov | |
Description | Data deposited in or computed by PubChem | |
Molecular Formula |
C13H22N4O3S |
Source
|
Source | PubChem | |
URL | https://pubchem.ncbi.nlm.nih.gov | |
Description | Data deposited in or computed by PubChem | |
Molecular Weight |
320.44 g/mol |
Source
|
Source | PubChem | |
URL | https://pubchem.ncbi.nlm.nih.gov | |
Description | Data deposited in or computed by PubChem | |
Disclaimer and Information on In-Vitro Research Products
Please be aware that all articles and product information presented on BenchChem are intended solely for informational purposes. The products available for purchase on BenchChem are specifically designed for in-vitro studies, which are conducted outside of living organisms. In-vitro studies, derived from the Latin term "in glass," involve experiments performed in controlled laboratory settings using cells or tissues. It is important to note that these products are not categorized as medicines or drugs, and they have not received approval from the FDA for the prevention, treatment, or cure of any medical condition, ailment, or disease. We must emphasize that any form of bodily introduction of these products into humans or animals is strictly prohibited by law. It is essential to adhere to these guidelines to ensure compliance with legal and ethical standards in research and experimentation.