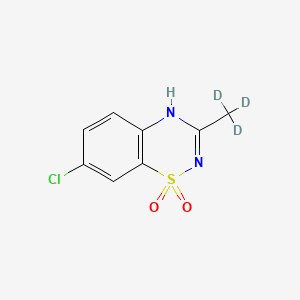
Diazoxide-d3
Overview
Description
Diazoxide-d3 is used as an internal standard for the quantification of diazoxide . Diazoxide is an activator of sulfonylurea receptor 1 (SUR1) linked to ATP-sensitive potassium channel K ir 6.2 . It also activates SUR2A/K ir 6.2 and SUR2B/K ir 6.2 channels in HEK293T cells . Diazoxide inhibits glucose-induced insulin release from isolated rat pancreatic β cells and induces relaxation of isolated rat aortic rings .
Molecular Structure Analysis
The molecular formula of Diazoxide-d3 is C8H4D3ClN2O2S . The average mass is 233.690 Da and the monoisotopic mass is 233.010498 Da .Chemical Reactions Analysis
Diazoxide is known to increase membrane permeability to potassium ions in vascular smooth muscle, thereby stabilizing the membrane action potential and preventing vascular smooth muscle contraction . This results in peripheral vasodilatation and decreases in peripheral vascular resistance .Scientific Research Applications
Multicomponent Reactions Involving Diazo Reagents
Diazoxide-d3 plays a significant role in multicomponent reactions of diazo compounds . These reactions are chemical transformations where three or more compounds react in a highly selective manner to form complex organic molecules . Diazoxide-d3, as a diazo compound, has unique and surprisingly diverse reactivity modes, including transformations with both the retention of nitrogen atoms and the elimination of dinitrogen during their thermal, catalytic, or photolytic activation .
Neuroprotection
Diazoxide-d3 has been shown to protect neurons from different neurotoxic insults in vitro and ex vivo . It effectively protects NSC-34 motoneurons from glutamatergic, oxidative, and inflammatory damage . This neuroprotective role of diazoxide is mediated by the activation of Nrf2 expression and nuclear translocation .
Antioxidative Pathway Activation
Diazoxide-d3 activates antioxidative pathways, which is a new mechanism of action that prevents neurodegeneration . This antioxidative pathway activation is beneficial for diseases such as multiple sclerosis .
ATP-Sensitive Potassium Channel Activation
Diazoxide-d3 is a well-known ATP-sensitive potassium channel activator . The activation of these channels has become a promising new therapeutic approach for the treatment of neurodegenerative diseases due to their role in mitochondrial and cellular protection .
Treatment of Hyperinsulinism
Diazoxide is the first-line drug for treating hyperinsulinism and is the only pharmacological agent approved for hyperinsulinism by the Federal Drug Administration . It’s worth noting that the deuterated form, Diazoxide-d3, would likely share this application due to its similar structure and function.
Synthesis of Natural Compounds
Diazo compounds like Diazoxide-d3 find their application in obtaining a variety of privileged motifs in drug design, such as a wide range of heterocyclic systems and spirocyclic scaffolds, as well as in the synthesis of natural compounds .
Safety And Hazards
Future Directions
properties
IUPAC Name |
7-chloro-3-(trideuteriomethyl)-4H-1λ6,2,4-benzothiadiazine 1,1-dioxide | |
---|---|---|
Source | PubChem | |
URL | https://pubchem.ncbi.nlm.nih.gov | |
Description | Data deposited in or computed by PubChem | |
InChI |
InChI=1S/C8H7ClN2O2S/c1-5-10-7-3-2-6(9)4-8(7)14(12,13)11-5/h2-4H,1H3,(H,10,11)/i1D3 | |
Source | PubChem | |
URL | https://pubchem.ncbi.nlm.nih.gov | |
Description | Data deposited in or computed by PubChem | |
InChI Key |
GDLBFKVLRPITMI-FIBGUPNXSA-N | |
Source | PubChem | |
URL | https://pubchem.ncbi.nlm.nih.gov | |
Description | Data deposited in or computed by PubChem | |
Canonical SMILES |
CC1=NS(=O)(=O)C2=C(N1)C=CC(=C2)Cl | |
Source | PubChem | |
URL | https://pubchem.ncbi.nlm.nih.gov | |
Description | Data deposited in or computed by PubChem | |
Isomeric SMILES |
[2H]C([2H])([2H])C1=NS(=O)(=O)C2=C(N1)C=CC(=C2)Cl | |
Source | PubChem | |
URL | https://pubchem.ncbi.nlm.nih.gov | |
Description | Data deposited in or computed by PubChem | |
Molecular Formula |
C8H7ClN2O2S | |
Source | PubChem | |
URL | https://pubchem.ncbi.nlm.nih.gov | |
Description | Data deposited in or computed by PubChem | |
Molecular Weight |
233.69 g/mol | |
Source | PubChem | |
URL | https://pubchem.ncbi.nlm.nih.gov | |
Description | Data deposited in or computed by PubChem | |
Product Name |
Diazoxide-d3 |
Retrosynthesis Analysis
AI-Powered Synthesis Planning: Our tool employs the Template_relevance Pistachio, Template_relevance Bkms_metabolic, Template_relevance Pistachio_ringbreaker, Template_relevance Reaxys, Template_relevance Reaxys_biocatalysis model, leveraging a vast database of chemical reactions to predict feasible synthetic routes.
One-Step Synthesis Focus: Specifically designed for one-step synthesis, it provides concise and direct routes for your target compounds, streamlining the synthesis process.
Accurate Predictions: Utilizing the extensive PISTACHIO, BKMS_METABOLIC, PISTACHIO_RINGBREAKER, REAXYS, REAXYS_BIOCATALYSIS database, our tool offers high-accuracy predictions, reflecting the latest in chemical research and data.
Strategy Settings
Precursor scoring | Relevance Heuristic |
---|---|
Min. plausibility | 0.01 |
Model | Template_relevance |
Template Set | Pistachio/Bkms_metabolic/Pistachio_ringbreaker/Reaxys/Reaxys_biocatalysis |
Top-N result to add to graph | 6 |
Feasible Synthetic Routes
Disclaimer and Information on In-Vitro Research Products
Please be aware that all articles and product information presented on BenchChem are intended solely for informational purposes. The products available for purchase on BenchChem are specifically designed for in-vitro studies, which are conducted outside of living organisms. In-vitro studies, derived from the Latin term "in glass," involve experiments performed in controlled laboratory settings using cells or tissues. It is important to note that these products are not categorized as medicines or drugs, and they have not received approval from the FDA for the prevention, treatment, or cure of any medical condition, ailment, or disease. We must emphasize that any form of bodily introduction of these products into humans or animals is strictly prohibited by law. It is essential to adhere to these guidelines to ensure compliance with legal and ethical standards in research and experimentation.