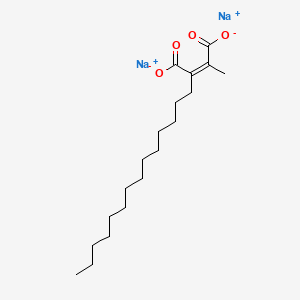
Chaetomellic Acid A Disodium Salt
Overview
Description
Mechanism of Action
Target of Action
Chaetomellic Acid A Disodium Salt, also known as disodium;(Z)-2-methyl-3-tetradecylbut-2-enedioate or Disodium (2Z)-2-methyl-3-tetradecylbut-2-enedioate, is a potent and highly specific inhibitor of Farnesyltransferase (FTase) . FTase is an enzyme that plays a crucial role in the post-translational modification of proteins, including the oncogenic protein Ha-Ras .
Mode of Action
This compound selectively blocks the farnesylation of Ha-Ras . Farnesylation is a critical step in the activation of Ha-Ras, which is involved in cell growth and differentiation. By inhibiting this process, this compound can potentially disrupt the function of Ha-Ras and its associated pathways .
Biochemical Pathways
The primary biochemical pathway affected by this compound is the Ras signaling pathway . By inhibiting FTase, the compound prevents the farnesylation and subsequent activation of Ha-Ras, thereby disrupting the Ras signaling pathway .
Result of Action
In a study involving a rat model of renal mass reduction, long-term administration of Chaetomellic Acid A was found to attenuate oxidative stress . This was evidenced by a significant increase in catalase and glutathione reductase activity, as well as an increase in the ratio of reduced glutathione to oxidized glutathione . No significant differences were found between the treated and non-treated groups in any of the parameters of renal function .
Biochemical Analysis
Biochemical Properties
Chaetomellic Acid A Disodium Salt plays a significant role in biochemical reactions. It acts as a potent inhibitor of farnesyl transferase in isolated enzyme assays . It is inactive in whole cells . The inhibition of farnesyltransferase is selective over geranylgeranyltransferase I and II . This compound interacts with enzymes such as farnesyl transferase, and its nature of interaction is inhibitory .
Cellular Effects
It is known that the compound has a significant impact on cell signaling pathways, gene expression, and cellular metabolism .
Molecular Mechanism
This compound exerts its effects at the molecular level primarily through its inhibitory action on farnesyl transferase . This inhibition leads to changes in gene expression and impacts the function of RAS proteins, which are involved in cell growth and differentiation .
Temporal Effects in Laboratory Settings
It is known that the compound is a potent inhibitor of farnesyl transferase in isolated enzyme assays
Metabolic Pathways
This compound is involved in the metabolic pathway of farnesylation, a type of protein prenylation . It interacts with the enzyme farnesyl transferase in this pathway .
Preparation Methods
Chaetomellic Acid A Disodium Salt can be synthesized through various methods. One common approach involves the reaction of chaetomellic acid with sodium hydroxide . Another practical route is based on the copper-catalyzed radical cyclization of (Z)-3-(2,2-dichloropropanoyl)-2-pentadecylidene-1,3-thiazinane . This method includes:
- A one-pot preparation of the intermediate N-α-perchloroacyl-2-(Z)-alkyliden-1,3-thiazinanes starting from N-(3-hydroxypropyl)palmitamide.
- A two-step smooth transformation of the radical cyclization products into chaetomellic acid.
- Only one intermediate chromatographic purification step .
Chemical Reactions Analysis
Chaetomellic Acid A Disodium Salt undergoes various chemical reactions, including:
Oxidation: This compound can be oxidized under specific conditions, leading to the formation of different oxidation products.
Reduction: It can also undergo reduction reactions, although specific conditions and reagents are required.
Substitution: This compound can participate in substitution reactions, where one functional group is replaced by another.
Common reagents and conditions used in these reactions include oxidizing agents, reducing agents, and catalysts. The major products formed from these reactions depend on the specific conditions and reagents used .
Scientific Research Applications
Chaetomellic Acid A Disodium Salt has several scientific research applications, including:
Comparison with Similar Compounds
Chaetomellic Acid A Disodium Salt can be compared with other similar compounds, such as:
Chaetomellic Acid B: Another inhibitor of farnesyl-protein transferase, but with different structural properties.
Farnesyl Diphosphate: A natural substrate for farnesyl-protein transferase, which this compound resembles in its inhibitory action.
The uniqueness of this compound lies in its specific structure, which allows it to act as a reversible inhibitor of farnesyl-protein transferase without affecting other related enzymes .
Properties
IUPAC Name |
disodium;(Z)-2-methyl-3-tetradecylbut-2-enedioate | |
---|---|---|
Source | PubChem | |
URL | https://pubchem.ncbi.nlm.nih.gov | |
Description | Data deposited in or computed by PubChem | |
InChI |
InChI=1S/C19H34O4.2Na/c1-3-4-5-6-7-8-9-10-11-12-13-14-15-17(19(22)23)16(2)18(20)21;;/h3-15H2,1-2H3,(H,20,21)(H,22,23);;/q;2*+1/p-2/b17-16-;; | |
Source | PubChem | |
URL | https://pubchem.ncbi.nlm.nih.gov | |
Description | Data deposited in or computed by PubChem | |
InChI Key |
FIKFWCKPWWTJBB-LSSNYKSTSA-L | |
Source | PubChem | |
URL | https://pubchem.ncbi.nlm.nih.gov | |
Description | Data deposited in or computed by PubChem | |
Canonical SMILES |
CCCCCCCCCCCCCCC(=C(C)C(=O)[O-])C(=O)[O-].[Na+].[Na+] | |
Source | PubChem | |
URL | https://pubchem.ncbi.nlm.nih.gov | |
Description | Data deposited in or computed by PubChem | |
Isomeric SMILES |
CCCCCCCCCCCCCC/C(=C(\C)/C(=O)[O-])/C(=O)[O-].[Na+].[Na+] | |
Source | PubChem | |
URL | https://pubchem.ncbi.nlm.nih.gov | |
Description | Data deposited in or computed by PubChem | |
Molecular Formula |
C19H32Na2O4 | |
Source | PubChem | |
URL | https://pubchem.ncbi.nlm.nih.gov | |
Description | Data deposited in or computed by PubChem | |
DSSTOX Substance ID |
DTXSID00747307 | |
Record name | Disodium (2Z)-2-methyl-3-tetradecylbut-2-enedioate | |
Source | EPA DSSTox | |
URL | https://comptox.epa.gov/dashboard/DTXSID00747307 | |
Description | DSSTox provides a high quality public chemistry resource for supporting improved predictive toxicology. | |
Molecular Weight |
370.4 g/mol | |
Source | PubChem | |
URL | https://pubchem.ncbi.nlm.nih.gov | |
Description | Data deposited in or computed by PubChem | |
CAS No. |
161308-35-6 | |
Record name | Disodium (2Z)-2-methyl-3-tetradecylbut-2-enedioate | |
Source | EPA DSSTox | |
URL | https://comptox.epa.gov/dashboard/DTXSID00747307 | |
Description | DSSTox provides a high quality public chemistry resource for supporting improved predictive toxicology. | |
Retrosynthesis Analysis
AI-Powered Synthesis Planning: Our tool employs the Template_relevance Pistachio, Template_relevance Bkms_metabolic, Template_relevance Pistachio_ringbreaker, Template_relevance Reaxys, Template_relevance Reaxys_biocatalysis model, leveraging a vast database of chemical reactions to predict feasible synthetic routes.
One-Step Synthesis Focus: Specifically designed for one-step synthesis, it provides concise and direct routes for your target compounds, streamlining the synthesis process.
Accurate Predictions: Utilizing the extensive PISTACHIO, BKMS_METABOLIC, PISTACHIO_RINGBREAKER, REAXYS, REAXYS_BIOCATALYSIS database, our tool offers high-accuracy predictions, reflecting the latest in chemical research and data.
Strategy Settings
Precursor scoring | Relevance Heuristic |
---|---|
Min. plausibility | 0.01 |
Model | Template_relevance |
Template Set | Pistachio/Bkms_metabolic/Pistachio_ringbreaker/Reaxys/Reaxys_biocatalysis |
Top-N result to add to graph | 6 |
Feasible Synthetic Routes
Disclaimer and Information on In-Vitro Research Products
Please be aware that all articles and product information presented on BenchChem are intended solely for informational purposes. The products available for purchase on BenchChem are specifically designed for in-vitro studies, which are conducted outside of living organisms. In-vitro studies, derived from the Latin term "in glass," involve experiments performed in controlled laboratory settings using cells or tissues. It is important to note that these products are not categorized as medicines or drugs, and they have not received approval from the FDA for the prevention, treatment, or cure of any medical condition, ailment, or disease. We must emphasize that any form of bodily introduction of these products into humans or animals is strictly prohibited by law. It is essential to adhere to these guidelines to ensure compliance with legal and ethical standards in research and experimentation.