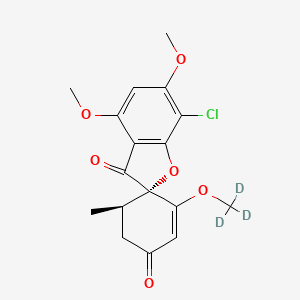
Griseofulvin-d3
Overview
Description
Griseofulvin-d3 is a deuterated form of griseofulvin, an antifungal agent primarily used to treat dermatophyte infections. The deuterium atoms in this compound replace three hydrogen atoms, making it useful as an internal standard in mass spectrometry for the quantification of griseofulvin .
Mechanism of Action
Target of Action
Griseofulvin-d3 primarily targets microtubules within fungal cells . Microtubules are key components of the cell’s cytoskeleton and play a crucial role in cell division . This compound binds to alpha and beta tubulin , the building blocks of microtubules .
Mode of Action
This compound is a fungistatic agent, meaning it inhibits the growth of fungi rather than killing them outright . It achieves this by disrupting the function of spindle and cytoplasmic microtubules . By binding to alpha and beta tubulin, this compound induces conformational changes that inhibit fungal cell mitosis and nuclear acid synthesis .
Biochemical Pathways
This compound affects the cell division cycle of fungal cells . By disrupting microtubule function, it interferes with the formation of the mitotic spindle, a structure that is essential for chromosome segregation during cell division . This disruption leads to cell cycle arrest at the G2/M phase, preventing the fungal cells from dividing and proliferating .
Pharmacokinetics
The pharmacokinetic properties of this compound have been improved through complexation with HP-γ-cyclodextrin . This complexation increases the water solubility of this compound by 477 times compared to this compound alone . In an in vivo dog pharmacokinetic study, the maximum concentration (Cmax) increased from 0.52 µg/mL to 0.72 µg/mL, and the area under the curve (AUC0–12) increased from 1.55 μg·h/mL to 2.75 μg·h/mL . The clearance changed from 51.78 L/kg/h to 24.16 L/kg/h, and the half-life time was extended from 0.81 h to 1.56 h .
Result of Action
The primary result of this compound’s action is the inhibition of fungal cell division, leading to a halt in the growth and proliferation of the fungus . This makes this compound an effective treatment for various fungal infections . Additionally, this compound has shown potential in disrupting mitosis and cell division in human cancer cells and arresting hepatitis C virus replication .
Action Environment
The action of this compound can be influenced by environmental factors. For instance, the complexation of this compound with HP-γ-cyclodextrin, a process facilitated by supercritical carbon dioxide, significantly improves its water solubility .
Biochemical Analysis
Biochemical Properties
Griseofulvin-d3 plays a significant role in biochemical reactions by interacting with various enzymes, proteins, and other biomolecules. It is known to bind to fungal microtubules, interfering with their function and inhibiting mitosis . This compound interacts with alpha and beta tubulin, which are essential components of the microtubule structure . This binding disrupts the polymerization of microtubules, leading to the inhibition of fungal cell division . Additionally, this compound has been shown to interact with cytokeratin intermediate filament proteins (K8 and K18), which may contribute to its effects on cellular structure and function .
Cellular Effects
This compound exerts various effects on different types of cells and cellular processes. In fungal cells, it disrupts mitosis by binding to microtubules and inhibiting their polymerization . This leads to the formation of multinucleate cells and ultimately cell death . In human cells, this compound has been shown to disrupt mitosis and cell division, particularly in cancer cells . It also influences cell signaling pathways, gene expression, and cellular metabolism by interacting with microtubules and other cellular structures . These effects can lead to changes in cell function and viability.
Molecular Mechanism
The molecular mechanism of action of this compound involves its binding interactions with biomolecules, enzyme inhibition, and changes in gene expression. This compound binds to alpha and beta tubulin, interfering with the function of spindle and cytoplasmic microtubules . This binding inhibits the polymerization of microtubules, preventing the formation of the mitotic spindle and leading to the inhibition of cell division . Additionally, this compound has been shown to inhibit the replication of hepatitis C virus by interfering with microtubule polymerization in human cells . These interactions at the molecular level contribute to the compound’s antifungal and anticancer properties.
Temporal Effects in Laboratory Settings
In laboratory settings, the effects of this compound change over time due to its stability, degradation, and long-term effects on cellular function. This compound is relatively stable, but its degradation products can interact with cellular structures and influence cellular function . Long-term studies have shown that this compound can lead to the formation of Mallory bodies in hepatocytes, which are associated with liver injury . Additionally, sustained release formulations of this compound have been developed to provide long-term therapeutic effects in preclinical models .
Dosage Effects in Animal Models
The effects of this compound vary with different dosages in animal models. At therapeutic doses, this compound is effective in treating dermatophyte infections in various animal species . At higher doses, it can cause toxic or adverse effects, including gastrointestinal upset, changes in blood cell production, and liver injury . The threshold effects observed in these studies indicate that careful dosage management is necessary to balance efficacy and safety.
Metabolic Pathways
This compound is involved in several metabolic pathways, including its interaction with enzymes and cofactors. The compound is metabolized by the liver, where it undergoes hydroxylation and demethylation to form active metabolites . These metabolites can interact with cytokeratin intermediate filament proteins, contributing to the compound’s effects on cellular structure and function . Additionally, this compound can influence metabolic flux and metabolite levels by interacting with enzymes involved in its metabolism .
Transport and Distribution
This compound is transported and distributed within cells and tissues through various mechanisms. It is absorbed from the gastrointestinal tract and distributed to keratin precursor cells, where it binds to keratin and persists in the stratum corneum . This binding makes it resistant to fungal invasion and allows for its therapeutic effects in treating dermatophyte infections . This compound is also transported through energy-dependent processes and can accumulate in specific cellular compartments .
Subcellular Localization
The subcellular localization of this compound is primarily within the microtubules and cytoskeletal structures of cells. It binds to alpha and beta tubulin, interfering with microtubule function and inhibiting mitosis . This localization is essential for its antifungal and anticancer properties, as it disrupts the formation of the mitotic spindle and prevents cell division . Additionally, this compound can interact with cytokeratin intermediate filament proteins, influencing cellular structure and function .
Preparation Methods
Synthetic Routes and Reaction Conditions
Griseofulvin-d3 can be synthesized through the deuteration of griseofulvin. The process involves the exchange of hydrogen atoms with deuterium atoms. This can be achieved using deuterated reagents under specific conditions. For example, griseofulvin can be treated with deuterated methanol (CD3OD) in the presence of a catalyst to facilitate the exchange .
Industrial Production Methods
Industrial production of this compound follows similar principles but on a larger scale. The process involves the use of deuterated solvents and catalysts to ensure the efficient exchange of hydrogen atoms with deuterium. The final product is then purified to achieve the desired level of deuteration and purity .
Chemical Reactions Analysis
Types of Reactions
Griseofulvin-d3 undergoes various chemical reactions, including:
Oxidation: this compound can be oxidized to form different metabolites.
Reduction: Reduction reactions can convert this compound into its reduced forms.
Substitution: Substitution reactions can occur at specific positions on the molecule, leading to the formation of various derivatives.
Common Reagents and Conditions
Oxidation: Common oxidizing agents include potassium permanganate (KMnO4) and hydrogen peroxide (H2O2).
Reduction: Reducing agents such as sodium borohydride (NaBH4) and lithium aluminum hydride (LiAlH4) are commonly used.
Substitution: Substitution reactions often involve halogenating agents like bromine (Br2) or chlorine (Cl2) under controlled conditions.
Major Products Formed
The major products formed from these reactions include various oxidized, reduced, and substituted derivatives of this compound. These derivatives can have different biological activities and properties .
Scientific Research Applications
Griseofulvin-d3 has a wide range of scientific research applications:
Chemistry: Used as an internal standard in mass spectrometry for the quantification of griseofulvin.
Biology: Studied for its effects on microtubule dynamics and cell division.
Medicine: Investigated for its potential use in cancer therapy due to its ability to disrupt mitosis.
Industry: Used in the development of antifungal formulations and as a reference standard in quality control
Comparison with Similar Compounds
Similar Compounds
Griseofulvin: The non-deuterated form of Griseofulvin-d3, used as an antifungal agent.
6-Desmethylgriseofulvin: A metabolite of griseofulvin with similar antifungal properties.
4-Desmethylgriseofulvin: Another metabolite with antifungal activity
Uniqueness
Griseofulvin-d3 is unique due to the presence of deuterium atoms, which makes it particularly useful as an internal standard in analytical chemistry. The deuterium atoms provide a distinct mass difference, allowing for precise quantification of griseofulvin in various samples .
Properties
IUPAC Name |
(2S,5'R)-7-chloro-4,6-dimethoxy-5'-methyl-3'-(trideuteriomethoxy)spiro[1-benzofuran-2,4'-cyclohex-2-ene]-1',3-dione | |
---|---|---|
Source | PubChem | |
URL | https://pubchem.ncbi.nlm.nih.gov | |
Description | Data deposited in or computed by PubChem | |
InChI |
InChI=1S/C17H17ClO6/c1-8-5-9(19)6-12(23-4)17(8)16(20)13-10(21-2)7-11(22-3)14(18)15(13)24-17/h6-8H,5H2,1-4H3/t8-,17+/m1/s1/i4D3 | |
Source | PubChem | |
URL | https://pubchem.ncbi.nlm.nih.gov | |
Description | Data deposited in or computed by PubChem | |
InChI Key |
DDUHZTYCFQRHIY-BCBJJHLGSA-N | |
Source | PubChem | |
URL | https://pubchem.ncbi.nlm.nih.gov | |
Description | Data deposited in or computed by PubChem | |
Canonical SMILES |
CC1CC(=O)C=C(C12C(=O)C3=C(O2)C(=C(C=C3OC)OC)Cl)OC | |
Source | PubChem | |
URL | https://pubchem.ncbi.nlm.nih.gov | |
Description | Data deposited in or computed by PubChem | |
Isomeric SMILES |
[2H]C([2H])([2H])OC1=CC(=O)C[C@H]([C@@]12C(=O)C3=C(O2)C(=C(C=C3OC)OC)Cl)C | |
Source | PubChem | |
URL | https://pubchem.ncbi.nlm.nih.gov | |
Description | Data deposited in or computed by PubChem | |
Molecular Formula |
C17H17ClO6 | |
Source | PubChem | |
URL | https://pubchem.ncbi.nlm.nih.gov | |
Description | Data deposited in or computed by PubChem | |
Molecular Weight |
355.8 g/mol | |
Source | PubChem | |
URL | https://pubchem.ncbi.nlm.nih.gov | |
Description | Data deposited in or computed by PubChem | |
Retrosynthesis Analysis
AI-Powered Synthesis Planning: Our tool employs the Template_relevance Pistachio, Template_relevance Bkms_metabolic, Template_relevance Pistachio_ringbreaker, Template_relevance Reaxys, Template_relevance Reaxys_biocatalysis model, leveraging a vast database of chemical reactions to predict feasible synthetic routes.
One-Step Synthesis Focus: Specifically designed for one-step synthesis, it provides concise and direct routes for your target compounds, streamlining the synthesis process.
Accurate Predictions: Utilizing the extensive PISTACHIO, BKMS_METABOLIC, PISTACHIO_RINGBREAKER, REAXYS, REAXYS_BIOCATALYSIS database, our tool offers high-accuracy predictions, reflecting the latest in chemical research and data.
Strategy Settings
Precursor scoring | Relevance Heuristic |
---|---|
Min. plausibility | 0.01 |
Model | Template_relevance |
Template Set | Pistachio/Bkms_metabolic/Pistachio_ringbreaker/Reaxys/Reaxys_biocatalysis |
Top-N result to add to graph | 6 |
Feasible Synthetic Routes
Disclaimer and Information on In-Vitro Research Products
Please be aware that all articles and product information presented on BenchChem are intended solely for informational purposes. The products available for purchase on BenchChem are specifically designed for in-vitro studies, which are conducted outside of living organisms. In-vitro studies, derived from the Latin term "in glass," involve experiments performed in controlled laboratory settings using cells or tissues. It is important to note that these products are not categorized as medicines or drugs, and they have not received approval from the FDA for the prevention, treatment, or cure of any medical condition, ailment, or disease. We must emphasize that any form of bodily introduction of these products into humans or animals is strictly prohibited by law. It is essential to adhere to these guidelines to ensure compliance with legal and ethical standards in research and experimentation.