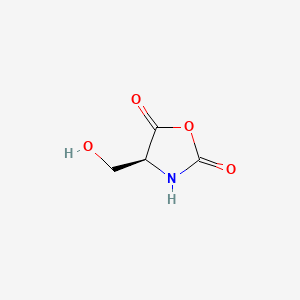
L-Serine N-Carboxyanhydride
Overview
Description
L-Serine N-Carboxyanhydride is a chemical compound that belongs to the class of oxazolidines. This compound is characterized by a five-membered ring containing both nitrogen and oxygen atoms. The presence of a hydroxymethyl group at the 4th position and a dione structure at the 2nd and 5th positions makes it a unique and interesting molecule for various scientific applications.
Mechanism of Action
Target of Action
L-Serine N-Carboxyanhydride (L-Serine NCA) primarily targets amino acids and peptides. It is used in the synthesis of polypeptides through ring-opening polymerization (ROP). The primary role of L-Serine NCA is to act as a monomer in the formation of polypeptides, which are essential for various biological functions and applications in biomaterials .
Mode of Action
L-Serine NCA interacts with nucleophiles, such as amines, initiating a ring-opening polymerization process. This interaction leads to the formation of polypeptides by linking amino acid units together. The ring-opening mechanism involves the nucleophilic attack on the carbonyl carbon of the NCA, resulting in the formation of a linear polypeptide chain and the release of carbon dioxide .
Biochemical Pathways
The primary biochemical pathway affected by L-Serine NCA is the synthesis of polypeptides. This pathway involves the polymerization of amino acids into long chains, which can then fold into specific structures to form functional proteins. The downstream effects include the formation of various secondary structures, such as alpha-helices and beta-sheets, which are crucial for the biological activity of proteins .
Pharmacokinetics
L-Serine NCA is moisture-sensitive and can hydrolyze to form the parent amino acid, L-serine, which may affect its stability and efficacy in biological systems .
Result of Action
The molecular and cellular effects of L-Serine NCA’s action include the formation of polypeptides with specific sequences and structures. These polypeptides can mimic natural proteins and have applications in drug delivery, tissue engineering, and other biomedical fields. The ability to control the polymerization process allows for the synthesis of polypeptides with desired properties and functionalities .
Action Environment
Environmental factors such as temperature, pH, and moisture significantly influence the action, efficacy, and stability of L-Serine NCA. High temperatures and moisture can lead to the hydrolysis of the compound, reducing its effectiveness in polymerization reactions. Optimal conditions for its use typically involve anhydrous environments and controlled temperatures to ensure efficient polymerization and stability .
This compound plays a crucial role in the synthesis of polypeptides, offering valuable applications in biomaterials and biomedical research. Understanding its mechanism of action and the factors influencing its stability can enhance its utility in various scientific and industrial fields.
: Amino acid N-carboxyanhydride - Wikipedia : Ring-Opening Polymerization of N-Carboxyanhydrides for Preparation of Polypeptides and Polypeptide-Based Hybrid Materials with Various Molecular Architectures | SpringerLink
Preparation Methods
Synthetic Routes and Reaction Conditions
The synthesis of L-Serine N-Carboxyanhydride typically involves the reaction of an appropriate amino alcohol with a carbonyl compound under acidic or basic conditions. One common method is the cyclization of N-(hydroxymethyl)amino acids in the presence of a dehydrating agent. The reaction conditions often include the use of solvents like dichloromethane or ethanol and catalysts such as p-toluenesulfonic acid.
Industrial Production Methods
Industrial production of this compound may involve continuous flow processes to ensure high yield and purity. The use of automated reactors and precise control of reaction parameters such as temperature, pressure, and pH are crucial for large-scale synthesis.
Chemical Reactions Analysis
Types of Reactions
L-Serine N-Carboxyanhydride undergoes various chemical reactions, including:
Oxidation: The hydroxymethyl group can be oxidized to form a carboxylic acid.
Reduction: The dione structure can be reduced to form a diol.
Substitution: The hydroxymethyl group can be substituted with other functional groups through nucleophilic substitution reactions.
Common Reagents and Conditions
Oxidation: Common oxidizing agents include potassium permanganate and chromium trioxide.
Reduction: Reducing agents such as lithium aluminum hydride or sodium borohydride are often used.
Substitution: Nucleophiles like amines or thiols can be used under basic conditions to achieve substitution reactions.
Major Products
Oxidation: Formation of (4S)-4-(carboxymethyl)-1,3-oxazolidine-2,5-dione.
Reduction: Formation of (4S)-4-(hydroxymethyl)-1,3-oxazolidine-2,5-diol.
Substitution: Formation of various substituted oxazolidines depending on the nucleophile used.
Scientific Research Applications
L-Serine N-Carboxyanhydride has a wide range of applications in scientific research:
Chemistry: Used as a building block for the synthesis of more complex molecules.
Biology: Studied for its potential role in enzyme inhibition and protein interactions.
Medicine: Investigated for its potential therapeutic effects, including antimicrobial and anticancer properties.
Industry: Utilized in the production of polymers and as a stabilizer in various formulations.
Comparison with Similar Compounds
Similar Compounds
- (4S)-4-(hydroxymethyl)-1,3-dioxolane-2,5-dione
- (4S)-4-(hydroxymethyl)-1,3-thiazolidine-2,5-dione
- (4S)-4-(hydroxymethyl)-1,3-oxazolidine-2-thione
Uniqueness
L-Serine N-Carboxyanhydride is unique due to its specific ring structure and the presence of both hydroxymethyl and dione functional groups. This combination allows for diverse chemical reactivity and a wide range of applications in various fields.
Properties
IUPAC Name |
(4S)-4-(hydroxymethyl)-1,3-oxazolidine-2,5-dione | |
---|---|---|
Source | PubChem | |
URL | https://pubchem.ncbi.nlm.nih.gov | |
Description | Data deposited in or computed by PubChem | |
InChI |
InChI=1S/C4H5NO4/c6-1-2-3(7)9-4(8)5-2/h2,6H,1H2,(H,5,8)/t2-/m0/s1 | |
Source | PubChem | |
URL | https://pubchem.ncbi.nlm.nih.gov | |
Description | Data deposited in or computed by PubChem | |
InChI Key |
KVUIJQRUDVLWAI-REOHCLBHSA-N | |
Source | PubChem | |
URL | https://pubchem.ncbi.nlm.nih.gov | |
Description | Data deposited in or computed by PubChem | |
Canonical SMILES |
C(C1C(=O)OC(=O)N1)O | |
Source | PubChem | |
URL | https://pubchem.ncbi.nlm.nih.gov | |
Description | Data deposited in or computed by PubChem | |
Isomeric SMILES |
C([C@H]1C(=O)OC(=O)N1)O | |
Source | PubChem | |
URL | https://pubchem.ncbi.nlm.nih.gov | |
Description | Data deposited in or computed by PubChem | |
Molecular Formula |
C4H5NO4 | |
Source | PubChem | |
URL | https://pubchem.ncbi.nlm.nih.gov | |
Description | Data deposited in or computed by PubChem | |
DSSTOX Substance ID |
DTXSID40717468 | |
Record name | (4S)-4-(Hydroxymethyl)-1,3-oxazolidine-2,5-dione | |
Source | EPA DSSTox | |
URL | https://comptox.epa.gov/dashboard/DTXSID40717468 | |
Description | DSSTox provides a high quality public chemistry resource for supporting improved predictive toxicology. | |
Molecular Weight |
131.09 g/mol | |
Source | PubChem | |
URL | https://pubchem.ncbi.nlm.nih.gov | |
Description | Data deposited in or computed by PubChem | |
CAS No. |
33043-54-8 | |
Record name | (4S)-4-(Hydroxymethyl)-1,3-oxazolidine-2,5-dione | |
Source | EPA DSSTox | |
URL | https://comptox.epa.gov/dashboard/DTXSID40717468 | |
Description | DSSTox provides a high quality public chemistry resource for supporting improved predictive toxicology. | |
Disclaimer and Information on In-Vitro Research Products
Please be aware that all articles and product information presented on BenchChem are intended solely for informational purposes. The products available for purchase on BenchChem are specifically designed for in-vitro studies, which are conducted outside of living organisms. In-vitro studies, derived from the Latin term "in glass," involve experiments performed in controlled laboratory settings using cells or tissues. It is important to note that these products are not categorized as medicines or drugs, and they have not received approval from the FDA for the prevention, treatment, or cure of any medical condition, ailment, or disease. We must emphasize that any form of bodily introduction of these products into humans or animals is strictly prohibited by law. It is essential to adhere to these guidelines to ensure compliance with legal and ethical standards in research and experimentation.