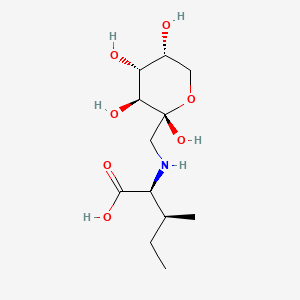
Fructose-isoleucine (mixture of diastereomers)
Overview
Description
Fructose-isoleucine is a compound formed by the conjugation of fructose, a monosaccharide, with isoleucine, a branched-chain amino acid. This compound exists as a mixture of diastereomers due to the presence of multiple chiral centers in both fructose and isoleucine. The combination of these two molecules results in a compound with unique properties that can be explored for various scientific and industrial applications.
Biochemical Analysis
Biochemical Properties
The role of N-(1-Deoxy-beta-D-fructopyranos-1-yl)-L-isoleucine in biochemical reactions is multifaceted. It interacts with a variety of enzymes, proteins, and other biomolecules . The nature of these interactions is complex and can vary depending on the specific context within the biochemical pathway.
Cellular Effects
N-(1-Deoxy-beta-D-fructopyranos-1-yl)-L-isoleucine influences cell function in several ways. It can impact cell signaling pathways, gene expression, and cellular metabolism . The specific effects can vary depending on the type of cell and the cellular context.
Molecular Mechanism
The mechanism of action of N-(1-Deoxy-beta-D-fructopyranos-1-yl)-L-isoleucine is complex. It can exert its effects at the molecular level through binding interactions with biomolecules, enzyme inhibition or activation, and changes in gene expression .
Temporal Effects in Laboratory Settings
In laboratory settings, the effects of N-(1-Deoxy-beta-D-fructopyranos-1-yl)-L-isoleucine can change over time. This includes information on the product’s stability, degradation, and any long-term effects on cellular function observed in in vitro or in vivo studies .
Dosage Effects in Animal Models
The effects of N-(1-Deoxy-beta-D-fructopyranos-1-yl)-L-isoleucine can vary with different dosages in animal models. This includes any threshold effects observed in these studies, as well as any toxic or adverse effects at high doses .
Metabolic Pathways
N-(1-Deoxy-beta-D-fructopyranos-1-yl)-L-isoleucine is involved in several metabolic pathways. It interacts with various enzymes and cofactors, and can have effects on metabolic flux or metabolite levels .
Transport and Distribution
N-(1-Deoxy-beta-D-fructopyranos-1-yl)-L-isoleucine is transported and distributed within cells and tissues in a complex manner. It interacts with various transporters and binding proteins, and can have effects on its localization or accumulation .
Subcellular Localization
The subcellular localization of N-(1-Deoxy-beta-D-fructopyranos-1-yl)-L-isoleucine can influence its activity or function. This includes any targeting signals or post-translational modifications that direct it to specific compartments or organelles .
Preparation Methods
Synthetic Routes and Reaction Conditions: The synthesis of fructose-isoleucine typically involves the coupling of fructose with isoleucine through a glycosidic bond formation. This can be achieved using enzymatic or chemical methods. Enzymatic synthesis often employs glycosyltransferases, which facilitate the transfer of the glycosyl group from a donor molecule to the amino acid. Chemical synthesis may involve the activation of the hydroxyl group of fructose using reagents such as trichloroacetonitrile, followed by coupling with isoleucine under basic conditions.
Industrial Production Methods: Industrial production of fructose-isoleucine can be scaled up using fermentation processes. Microorganisms such as Escherichia coli can be genetically engineered to express enzymes that catalyze the formation of fructose-isoleucine. The fermentation process involves the cultivation of these microorganisms in bioreactors, followed by the extraction and purification of the desired compound.
Chemical Reactions Analysis
Types of Reactions: Fructose-isoleucine can undergo various chemical reactions, including:
Oxidation: The hydroxyl groups of fructose can be oxidized to form carbonyl compounds.
Reduction: The carbonyl groups can be reduced to form alcohols.
Substitution: The glycosidic bond can be hydrolyzed under acidic or basic conditions to release fructose and isoleucine.
Common Reagents and Conditions:
Oxidation: Reagents such as potassium permanganate or chromium trioxide can be used under acidic conditions.
Reduction: Sodium borohydride or lithium aluminum hydride can be used under mild conditions.
Substitution: Hydrochloric acid or sodium hydroxide can be used to hydrolyze the glycosidic bond.
Major Products:
Oxidation: Formation of fructose-derived carbonyl compounds.
Reduction: Formation of reduced fructose derivatives.
Substitution: Release of free fructose and isoleucine.
Scientific Research Applications
Fructose-isoleucine has several scientific research applications, including:
Chemistry: Used as a model compound to study glycosidic bond formation and cleavage.
Biology: Investigated for its role in metabolic pathways involving fructose and branched-chain amino acids.
Medicine: Explored for its potential effects on insulin secretion and glucose metabolism.
Industry: Utilized in the production of functional foods and dietary supplements.
Mechanism of Action
The mechanism of action of fructose-isoleucine involves its interaction with metabolic pathways related to fructose and isoleucine. Fructose is known to influence insulin secretion and glucose metabolism, while isoleucine plays a role in protein synthesis and energy regulation. The combined effects of these molecules may enhance their individual metabolic impacts, potentially leading to improved glucose homeostasis and energy balance.
Comparison with Similar Compounds
Fructose-isoleucine can be compared with other similar compounds, such as:
4-Hydroxyisoleucine: Found in fenugreek seeds, known for its glucose-lowering effects.
Leucine: Another branched-chain amino acid with similar metabolic roles.
Valine: A branched-chain amino acid involved in protein synthesis and energy production.
Uniqueness: Fructose-isoleucine is unique due to its combination of a monosaccharide and a branched-chain amino acid, which allows it to participate in both carbohydrate and amino acid metabolism. This dual functionality makes it a valuable compound for studying metabolic interactions and developing functional foods.
Properties
IUPAC Name |
(2S,3S)-3-methyl-2-[[(2R,3S,4R,5R)-2,3,4,5-tetrahydroxyoxan-2-yl]methylamino]pentanoic acid | |
---|---|---|
Source | PubChem | |
URL | https://pubchem.ncbi.nlm.nih.gov | |
Description | Data deposited in or computed by PubChem | |
InChI |
InChI=1S/C12H23NO7/c1-3-6(2)8(11(17)18)13-5-12(19)10(16)9(15)7(14)4-20-12/h6-10,13-16,19H,3-5H2,1-2H3,(H,17,18)/t6-,7+,8-,9+,10-,12+/m0/s1 | |
Source | PubChem | |
URL | https://pubchem.ncbi.nlm.nih.gov | |
Description | Data deposited in or computed by PubChem | |
InChI Key |
ADLSFIUFBSPKJF-KZYWSPAVSA-N | |
Source | PubChem | |
URL | https://pubchem.ncbi.nlm.nih.gov | |
Description | Data deposited in or computed by PubChem | |
Canonical SMILES |
CCC(C)C(C(=O)O)NCC1(C(C(C(CO1)O)O)O)O | |
Source | PubChem | |
URL | https://pubchem.ncbi.nlm.nih.gov | |
Description | Data deposited in or computed by PubChem | |
Isomeric SMILES |
CC[C@H](C)[C@@H](C(=O)O)NC[C@@]1([C@H]([C@@H]([C@@H](CO1)O)O)O)O | |
Source | PubChem | |
URL | https://pubchem.ncbi.nlm.nih.gov | |
Description | Data deposited in or computed by PubChem | |
Molecular Formula |
C12H23NO7 | |
Source | PubChem | |
URL | https://pubchem.ncbi.nlm.nih.gov | |
Description | Data deposited in or computed by PubChem | |
DSSTOX Substance ID |
DTXSID101242010 | |
Record name | N-(1-Deoxy-β-D-fructopyranos-1-yl)-L-isoleucine | |
Source | EPA DSSTox | |
URL | https://comptox.epa.gov/dashboard/DTXSID101242010 | |
Description | DSSTox provides a high quality public chemistry resource for supporting improved predictive toxicology. | |
Molecular Weight |
293.31 g/mol | |
Source | PubChem | |
URL | https://pubchem.ncbi.nlm.nih.gov | |
Description | Data deposited in or computed by PubChem | |
CAS No. |
87304-79-8 | |
Record name | N-(1-Deoxy-β-D-fructopyranos-1-yl)-L-isoleucine | |
Source | CAS Common Chemistry | |
URL | https://commonchemistry.cas.org/detail?cas_rn=87304-79-8 | |
Description | CAS Common Chemistry is an open community resource for accessing chemical information. Nearly 500,000 chemical substances from CAS REGISTRY cover areas of community interest, including common and frequently regulated chemicals, and those relevant to high school and undergraduate chemistry classes. This chemical information, curated by our expert scientists, is provided in alignment with our mission as a division of the American Chemical Society. | |
Explanation | The data from CAS Common Chemistry is provided under a CC-BY-NC 4.0 license, unless otherwise stated. | |
Record name | N-(1-Deoxy-β-D-fructopyranos-1-yl)-L-isoleucine | |
Source | EPA DSSTox | |
URL | https://comptox.epa.gov/dashboard/DTXSID101242010 | |
Description | DSSTox provides a high quality public chemistry resource for supporting improved predictive toxicology. | |
Disclaimer and Information on In-Vitro Research Products
Please be aware that all articles and product information presented on BenchChem are intended solely for informational purposes. The products available for purchase on BenchChem are specifically designed for in-vitro studies, which are conducted outside of living organisms. In-vitro studies, derived from the Latin term "in glass," involve experiments performed in controlled laboratory settings using cells or tissues. It is important to note that these products are not categorized as medicines or drugs, and they have not received approval from the FDA for the prevention, treatment, or cure of any medical condition, ailment, or disease. We must emphasize that any form of bodily introduction of these products into humans or animals is strictly prohibited by law. It is essential to adhere to these guidelines to ensure compliance with legal and ethical standards in research and experimentation.