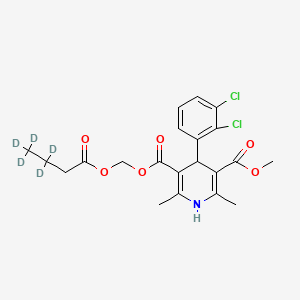
Clevidipine-d5
Overview
Description
Clevidipine-d5 is a deuterated form of clevidipine, a third-generation ultra-short-acting dihydropyridine calcium channel blocker. It is primarily used for the rapid reduction of blood pressure in situations where oral antihypertensive therapy is not feasible or desirable. This compound is structurally similar to clevidipine but contains five deuterium atoms, which can be useful in pharmacokinetic studies to differentiate it from the non-deuterated form .
Preparation Methods
Synthetic Routes and Reaction Conditions
The synthesis of clevidipine-d5 involves the incorporation of deuterium atoms into the clevidipine molecule This can be achieved through various methods, including the use of deuterated reagents or solvents during the synthesis processThe reaction conditions typically involve the use of catalysts, controlled temperatures, and specific solvents to ensure the selective incorporation of deuterium .
Industrial Production Methods
Industrial production of this compound follows similar principles as the laboratory synthesis but on a larger scale. The process involves the use of high-purity deuterated reagents and solvents, as well as advanced purification techniques to ensure the final product meets the required specifications. The production process is designed to be efficient and cost-effective, with a focus on minimizing waste and maximizing yield .
Chemical Reactions Analysis
Types of Reactions
Clevidipine-d5 undergoes various chemical reactions, including:
Oxidation: this compound can be oxidized to form corresponding carboxylic acids.
Reduction: Reduction reactions can convert this compound back to its dihydropyridine form.
Substitution: This compound can undergo substitution reactions where one or more of its functional groups are replaced by other groups
Common Reagents and Conditions
Oxidation: Common oxidizing agents include potassium permanganate and chromium trioxide.
Reduction: Reducing agents such as lithium aluminum hydride and sodium borohydride are commonly used.
Substitution: Various nucleophiles and electrophiles can be used in substitution reactions, depending on the desired product
Major Products Formed
The major products formed from these reactions include carboxylic acids, reduced dihydropyridine derivatives, and substituted this compound analogs .
Scientific Research Applications
Clevidipine-d5 has several scientific research applications, including:
Pharmacokinetic Studies: The deuterium atoms in this compound allow researchers to track its metabolism and distribution in the body more accurately.
Drug Development: this compound is used in the development of new antihypertensive drugs and to study the effects of deuterium substitution on drug efficacy and safety.
Biological Research: this compound is used to investigate the mechanisms of calcium channel blockers and their effects on vascular smooth muscle cells.
Medical Research: This compound is studied for its potential use in treating acute hypertension and other cardiovascular conditions .
Mechanism of Action
Clevidipine-d5 works by selectively inhibiting L-type calcium channels in vascular smooth muscle cells. This inhibition prevents the influx of calcium ions, leading to the relaxation of the smooth muscle cells and subsequent vasodilation. The reduction in systemic vascular resistance results in a decrease in blood pressure. The deuterium atoms in this compound do not significantly alter its mechanism of action compared to the non-deuterated form .
Comparison with Similar Compounds
Clevidipine-d5 is similar to other dihydropyridine calcium channel blockers, such as:
Nicardipine: Both this compound and nicardipine are used to treat hypertension, but this compound has a shorter half-life and faster onset of action.
Amlodipine: Amlodipine is another calcium channel blocker with a longer duration of action compared to this compound.
Nifedipine: Nifedipine is used for chronic hypertension management, whereas this compound is used for acute blood pressure reduction .
This compound’s uniqueness lies in its ultra-short half-life and rapid metabolism by esterases, making it suitable for acute settings where rapid blood pressure control is needed .
Properties
IUPAC Name |
3-O-methyl 5-O-(3,3,4,4,4-pentadeuteriobutanoyloxymethyl) 4-(2,3-dichlorophenyl)-2,6-dimethyl-1,4-dihydropyridine-3,5-dicarboxylate | |
---|---|---|
Source | PubChem | |
URL | https://pubchem.ncbi.nlm.nih.gov | |
Description | Data deposited in or computed by PubChem | |
InChI |
InChI=1S/C21H23Cl2NO6/c1-5-7-15(25)29-10-30-21(27)17-12(3)24-11(2)16(20(26)28-4)18(17)13-8-6-9-14(22)19(13)23/h6,8-9,18,24H,5,7,10H2,1-4H3/i1D3,5D2 | |
Source | PubChem | |
URL | https://pubchem.ncbi.nlm.nih.gov | |
Description | Data deposited in or computed by PubChem | |
InChI Key |
KPBZROQVTHLCDU-RPIBLTHZSA-N | |
Source | PubChem | |
URL | https://pubchem.ncbi.nlm.nih.gov | |
Description | Data deposited in or computed by PubChem | |
Canonical SMILES |
CCCC(=O)OCOC(=O)C1=C(NC(=C(C1C2=C(C(=CC=C2)Cl)Cl)C(=O)OC)C)C | |
Source | PubChem | |
URL | https://pubchem.ncbi.nlm.nih.gov | |
Description | Data deposited in or computed by PubChem | |
Isomeric SMILES |
[2H]C([2H])([2H])C([2H])([2H])CC(=O)OCOC(=O)C1=C(NC(=C(C1C2=C(C(=CC=C2)Cl)Cl)C(=O)OC)C)C | |
Source | PubChem | |
URL | https://pubchem.ncbi.nlm.nih.gov | |
Description | Data deposited in or computed by PubChem | |
Molecular Formula |
C21H23Cl2NO6 | |
Source | PubChem | |
URL | https://pubchem.ncbi.nlm.nih.gov | |
Description | Data deposited in or computed by PubChem | |
Molecular Weight |
461.3 g/mol | |
Source | PubChem | |
URL | https://pubchem.ncbi.nlm.nih.gov | |
Description | Data deposited in or computed by PubChem | |
Synthesis routes and methods
Procedure details
Disclaimer and Information on In-Vitro Research Products
Please be aware that all articles and product information presented on BenchChem are intended solely for informational purposes. The products available for purchase on BenchChem are specifically designed for in-vitro studies, which are conducted outside of living organisms. In-vitro studies, derived from the Latin term "in glass," involve experiments performed in controlled laboratory settings using cells or tissues. It is important to note that these products are not categorized as medicines or drugs, and they have not received approval from the FDA for the prevention, treatment, or cure of any medical condition, ailment, or disease. We must emphasize that any form of bodily introduction of these products into humans or animals is strictly prohibited by law. It is essential to adhere to these guidelines to ensure compliance with legal and ethical standards in research and experimentation.