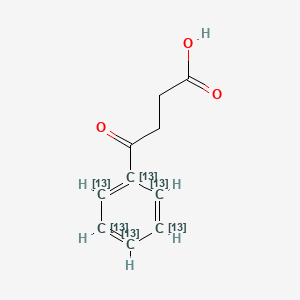
4-((1,2,3,4,5,6-13C6)cyclohexatrienyl)-4-oxobutanoic acid
Overview
Description
4-((1,2,3,4,5,6-13C6)cyclohexatrienyl)-4-oxobutanoic acid is an isotopically labeled compound where six carbon atoms are replaced with the stable isotope carbon-13. This compound is a derivative of 3-Benzoylpropanoic Acid, which is known for its applications in organic synthesis and as a non-steroidal anti-inflammatory drug (NSAID) used to treat pain, fever, and inflammation. The isotopic labeling makes it particularly useful in various scientific research fields, including mass spectrometry and metabolic studies .
Preparation Methods
Synthetic Routes and Reaction Conditions: 4-((1,2,3,4,5,6-13C6)cyclohexatrienyl)-4-oxobutanoic acid can be synthesized using several methods. One common method involves the reaction of succinic anhydride with benzene in the presence of anhydrous aluminum chloride . The reaction mixture is heated and then treated with water and hydrochloric acid to precipitate the product. Another method involves the use of phenyl-magnesium bromide with succinic anhydride .
Industrial Production Methods: Industrial production of this compound typically involves large-scale synthesis using similar methods but optimized for higher yields and purity. The use of automated reactors and stringent quality control measures ensures the consistent production of high-quality isotopically labeled compounds.
Chemical Reactions Analysis
Types of Reactions: 4-((1,2,3,4,5,6-13C6)cyclohexatrienyl)-4-oxobutanoic acid undergoes various chemical reactions, including oxidation, reduction, and substitution reactions.
Common Reagents and Conditions:
Oxidation: Oxidation of this compound can be carried out using N-chlorobenzamide in an aqueous acetic acid medium.
Reduction: Reduction reactions typically involve the use of reducing agents like sodium borohydride or lithium aluminum hydride.
Substitution: Substitution reactions can be performed using halogenating agents or nucleophiles under appropriate conditions.
Major Products: The major products formed from these reactions include carboxylic acids, alcohols, and substituted benzoyl derivatives.
Scientific Research Applications
4-((1,2,3,4,5,6-13C6)cyclohexatrienyl)-4-oxobutanoic acid has a wide range of applications in scientific research:
Chemistry: Used as a reference standard in nuclear magnetic resonance (NMR) spectroscopy and mass spectrometry for studying reaction mechanisms and kinetics.
Biology: Employed in metabolic studies to trace biochemical pathways and understand metabolic fluxes.
Medicine: Utilized in drug development and pharmacokinetic studies to investigate the absorption, distribution, metabolism, and excretion of drugs.
Industry: Applied in the synthesis of complex organic molecules and as a precursor in the production of pharmaceuticals and fine chemicals.
Mechanism of Action
The mechanism of action of 4-((1,2,3,4,5,6-13C6)cyclohexatrienyl)-4-oxobutanoic acid involves its interaction with various molecular targets and pathways. In oxidation reactions, for example, the compound reacts with oxidizing agents to form carboxylic acids, with the rate of reaction influenced by factors such as the concentration of reactants and the dielectric constant of the medium . The isotopic labeling allows for precise tracking of the compound in metabolic studies, providing insights into its biochemical interactions and pathways.
Comparison with Similar Compounds
Comparison: 4-((1,2,3,4,5,6-13C6)cyclohexatrienyl)-4-oxobutanoic acid is unique due to its isotopic labeling, which enhances its utility in research applications requiring precise tracking and quantification. While similar compounds like 3-Benzoylpropionic Acid share some chemical properties, the presence of carbon-13 in this compound provides a distinct advantage in studies involving isotopic labeling and mass spectrometry .
Properties
IUPAC Name |
4-((1,2,3,4,5,6-13C6)cyclohexatrienyl)-4-oxobutanoic acid | |
---|---|---|
Source | PubChem | |
URL | https://pubchem.ncbi.nlm.nih.gov | |
Description | Data deposited in or computed by PubChem | |
InChI |
InChI=1S/C10H10O3/c11-9(6-7-10(12)13)8-4-2-1-3-5-8/h1-5H,6-7H2,(H,12,13)/i1+1,2+1,3+1,4+1,5+1,8+1 | |
Source | PubChem | |
URL | https://pubchem.ncbi.nlm.nih.gov | |
Description | Data deposited in or computed by PubChem | |
InChI Key |
KMQLIDDEQAJAGJ-DEHIIRIRSA-N | |
Source | PubChem | |
URL | https://pubchem.ncbi.nlm.nih.gov | |
Description | Data deposited in or computed by PubChem | |
Canonical SMILES |
C1=CC=C(C=C1)C(=O)CCC(=O)O | |
Source | PubChem | |
URL | https://pubchem.ncbi.nlm.nih.gov | |
Description | Data deposited in or computed by PubChem | |
Isomeric SMILES |
[13CH]1=[13CH][13CH]=[13C]([13CH]=[13CH]1)C(=O)CCC(=O)O | |
Source | PubChem | |
URL | https://pubchem.ncbi.nlm.nih.gov | |
Description | Data deposited in or computed by PubChem | |
Molecular Formula |
C10H10O3 | |
Source | PubChem | |
URL | https://pubchem.ncbi.nlm.nih.gov | |
Description | Data deposited in or computed by PubChem | |
Molecular Weight |
184.14 g/mol | |
Source | PubChem | |
URL | https://pubchem.ncbi.nlm.nih.gov | |
Description | Data deposited in or computed by PubChem | |
CAS No. |
1346600-75-6 | |
Record name | ||
Source | CAS Common Chemistry | |
URL | https://commonchemistry.cas.org/detail?cas_rn=1346600-75-6 | |
Description | CAS Common Chemistry is an open community resource for accessing chemical information. Nearly 500,000 chemical substances from CAS REGISTRY cover areas of community interest, including common and frequently regulated chemicals, and those relevant to high school and undergraduate chemistry classes. This chemical information, curated by our expert scientists, is provided in alignment with our mission as a division of the American Chemical Society. | |
Explanation | The data from CAS Common Chemistry is provided under a CC-BY-NC 4.0 license, unless otherwise stated. | |
Synthesis routes and methods I
Procedure details
Synthesis routes and methods II
Procedure details
Disclaimer and Information on In-Vitro Research Products
Please be aware that all articles and product information presented on BenchChem are intended solely for informational purposes. The products available for purchase on BenchChem are specifically designed for in-vitro studies, which are conducted outside of living organisms. In-vitro studies, derived from the Latin term "in glass," involve experiments performed in controlled laboratory settings using cells or tissues. It is important to note that these products are not categorized as medicines or drugs, and they have not received approval from the FDA for the prevention, treatment, or cure of any medical condition, ailment, or disease. We must emphasize that any form of bodily introduction of these products into humans or animals is strictly prohibited by law. It is essential to adhere to these guidelines to ensure compliance with legal and ethical standards in research and experimentation.