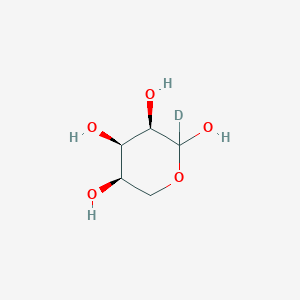
d-Ribose-1-d
- Click on QUICK INQUIRY to receive a quote from our team of experts.
- With the quality product at a COMPETITIVE price, you can focus more on your research.
Overview
Description
d-Ribose-1-d: is a naturally occurring sugar molecule that plays a crucial role in the production of adenosine triphosphate, which is the primary source of energy for cells. It is classified as a monosaccharide, meaning it is the simplest form of sugar and cannot be further broken down into smaller units. This compound is found in abundance in various living organisms, including plants, animals, and microorganisms . It is particularly important in the context of nutrition and supplements because it has been associated with enhancing energy levels and improving athletic performance .
Mechanism of Action
Target of Action
D-[1-2H]ribose, also known as d-ribose-1-d or this compound, primarily targets the enzyme Decaprenyl-phosphoryl-ribose 2′-epimerase (DprE1) . DprE1 is associated with a vital step of epimerisation in mycobacterial cell wall biosynthesis . It also targets the TRPM2 ion channel .
Mode of Action
D-[1-2H]ribose interacts with its targets and induces changes in their function. It is active in glycation, leading to the rapid production of advanced glycation end products (AGEs) in vitro and in vivo . It also activates central carbon metabolism, including glycolysis, the pentose phosphate pathway (PPP), and the tricarboxylic acid cycle (TCA cycle), increases the abundance of NADH, polarizes the electron transport chain (ETC), and elevates the proton motive force (PMF) of cells .
Biochemical Pathways
D-[1-2H]ribose is involved in several biochemical pathways. It is a component of the D-ribose Transport Pathway, which is researched in relation to transport . It also participates in the pentose phosphate pathway (PPP), contributing to adenosine triphosphate (ATP) production . Supplemental D-ribose can bypass part of the PPP, providing an alternate source of 5-phospho-d-ribose 1-pyrophosphate for ATP production .
Pharmacokinetics
D-[1-2H]ribose is rapidly absorbed with a mean Tmax ranging between 18 and 30 minutes . It rapidly disappears from plasma within less than 140 minutes . D-[1-2H]ribose follows a dose-dependent kinetic profile, with clearance seeming to follow first-order kinetics at low doses . It is partially recovered from urine, indicating it undergoes several metabolic pathways .
Result of Action
The action of D-[1-2H]ribose leads to various molecular and cellular effects. It induces protein aggregation, rapidly producing AGEs in vitro and in vivo . This ribosylation may be involved in cell dysfunction and subsequent cognitive impairments . It also enhances the recovery of ATP levels while reducing cellular injury .
Action Environment
The action of D-[1-2H]ribose can be influenced by environmental factors. For instance, the extracellular phosphate concentration and dissolved oxygen concentration can affect the flux through the PPP . Moreover, research showed that 10 µM–5 mM D-ribose could inhibit the extracellular polymeric substance (EPS) secretion of E. coli .
Biochemical Analysis
Biochemical Properties
D-[1-2H]ribose plays a crucial role in several biochemical reactions. It is a key component in the pentose phosphate pathway, where it is converted into ribose-5-phosphate. This compound interacts with several enzymes, including ribokinase, which phosphorylates d-ribose to form ribose-5-phosphate . Additionally, D-[1-2H]ribose is involved in the synthesis of nucleotides and nucleic acids, interacting with enzymes such as ribose-phosphate diphosphokinase . These interactions are essential for the production of ATP, NADH, and other critical biomolecules.
Cellular Effects
D-[1-2H]ribose influences various cellular processes. It enhances ATP production, which is vital for cellular energy metabolism . This compound also affects cell signaling pathways and gene expression. For instance, it has been shown to modulate the activity of transcription factors and enzymes involved in oxidative stress responses . In certain cell types, exogenous D-[1-2H]ribose can induce apoptosis, highlighting its potential impact on cell viability and function .
Molecular Mechanism
At the molecular level, D-[1-2H]ribose exerts its effects through several mechanisms. It binds to and activates ribokinase, facilitating the conversion of d-ribose to ribose-5-phosphate . This activation is crucial for the pentose phosphate pathway and subsequent nucleotide synthesis. Additionally, D-[1-2H]ribose can undergo non-enzymatic glycation, forming advanced glycation end products (AGEs) that can alter protein function and gene expression . These molecular interactions underscore the compound’s role in cellular metabolism and stress responses.
Temporal Effects in Laboratory Settings
In laboratory settings, the effects of D-[1-2H]ribose can vary over time. Studies have shown that this compound is relatively stable under physiological conditions but can degrade over extended periods . Long-term exposure to D-[1-2H]ribose in vitro has been associated with changes in cellular function, including alterations in metabolic activity and increased formation of AGEs . These temporal effects are important for understanding the compound’s stability and long-term impact on cellular processes.
Dosage Effects in Animal Models
The effects of D-[1-2H]ribose vary with different dosages in animal models. Low doses of this compound have been shown to enhance cardiac function and improve energy metabolism . High doses can lead to adverse effects, including oxidative stress and tissue damage . These dosage-dependent effects highlight the importance of careful dosing in experimental and therapeutic applications of D-[1-2H]ribose.
Metabolic Pathways
D-[1-2H]ribose is involved in several metabolic pathways, primarily the pentose phosphate pathway . In this pathway, it is converted to ribose-5-phosphate by ribokinase, which then participates in nucleotide synthesis and energy production . Additionally, D-[1-2H]ribose can be metabolized through glycolysis and the tricarboxylic acid cycle, contributing to overall cellular energy metabolism . These pathways underscore the compound’s central role in cellular biochemistry.
Transport and Distribution
Within cells and tissues, D-[1-2H]ribose is transported and distributed through specific transporters and binding proteins . For example, GLUT2 has been identified as a transporter that facilitates the uptake of D-[1-2H]ribose into hepatic cells . Once inside the cell, D-[1-2H]ribose can be phosphorylated and incorporated into various metabolic pathways. Its distribution within tissues is influenced by factors such as blood flow and tissue-specific transporter expression .
Subcellular Localization
D-[1-2H]ribose is localized in various subcellular compartments, including the cytoplasm and mitochondria . Its activity and function are influenced by its localization, with different effects observed in distinct cellular compartments. For instance, in the cytoplasm, D-[1-2H]ribose participates in the pentose phosphate pathway, while in the mitochondria, it can influence energy production and oxidative stress responses . The subcellular localization of D-[1-2H]ribose is critical for its diverse biochemical roles.
Preparation Methods
Synthetic Routes and Reaction Conditions:
Microbial Production: d-Ribose-1-d can be produced through microbial fermentation processes using bacteria such as Bacillus species.
Chemical Synthesis: Another method involves the cyanohydrin synthesis starting from d-erythrose.
Industrial Production Methods:
Fermentation: Large-scale production of this compound is typically achieved through fermentation processes using genetically engineered strains of bacteria or yeast.
Enzymatic Conversion: Enzymes such as ribokinase and ribose-5-phosphate isomerase can be used to convert ribose-5-phosphate into this compound.
Chemical Reactions Analysis
Types of Reactions:
Oxidation: d-Ribose-1-d can undergo oxidation reactions to form ribonic acid.
Reduction: Reduction of this compound can yield ribitol, a sugar alcohol.
Common Reagents and Conditions:
Oxidation: Nitric acid, potassium permanganate, and other strong oxidizing agents.
Reduction: Sodium borohydride, lithium aluminum hydride.
Substitution: Acidic conditions, nitrogenous bases like adenine, guanine, cytosine, and thymine.
Major Products:
Oxidation: Ribonic acid.
Reduction: Ribitol.
Substitution: Nucleosides such as adenosine, guanosine, cytidine, and thymidine.
Scientific Research Applications
Chemistry:
- d-Ribose-1-d is used as a precursor in the synthesis of various nucleotides and nucleosides, which are essential components of DNA and RNA .
Biology:
- It plays a vital role in cellular metabolism and energy production. This compound is a key component in the synthesis of adenosine triphosphate, which is crucial for muscle contraction and overall cellular function .
Medicine:
- This compound supplementation has been studied for its potential benefits in treating conditions such as chronic fatigue syndrome, fibromyalgia, and heart disease. It may help improve energy levels, reduce symptoms of fatigue, and enhance overall well-being .
Industry:
Comparison with Similar Compounds
d-Xylose: Another five-carbon sugar that is structurally similar to d-Ribose-1-d but differs in the arrangement of hydroxyl groups.
d-Arabinose: A pentose sugar that is an epimer of this compound at the second carbon atom.
d-Lyxose: Another aldopentose that differs from this compound in the configuration of hydroxyl groups.
Uniqueness:
- This compound is unique due to its specific role in the synthesis of adenosine triphosphate, making it a valuable compound in the field of nutrition and supplements . Unlike other pentose sugars, this compound is directly involved in energy production and has been associated with enhancing athletic performance and improving recovery from intense physical activity .
Properties
IUPAC Name |
(3R,4R,5R)-2-deuteriooxane-2,3,4,5-tetrol |
Source
|
---|---|---|
Source | PubChem | |
URL | https://pubchem.ncbi.nlm.nih.gov | |
Description | Data deposited in or computed by PubChem | |
InChI |
InChI=1S/C5H10O5/c6-2-1-10-5(9)4(8)3(2)7/h2-9H,1H2/t2-,3-,4-,5?/m1/s1/i5D |
Source
|
Source | PubChem | |
URL | https://pubchem.ncbi.nlm.nih.gov | |
Description | Data deposited in or computed by PubChem | |
InChI Key |
SRBFZHDQGSBBOR-ROIYBNBJSA-N |
Source
|
Source | PubChem | |
URL | https://pubchem.ncbi.nlm.nih.gov | |
Description | Data deposited in or computed by PubChem | |
Canonical SMILES |
C1C(C(C(C(O1)O)O)O)O |
Source
|
Source | PubChem | |
URL | https://pubchem.ncbi.nlm.nih.gov | |
Description | Data deposited in or computed by PubChem | |
Isomeric SMILES |
[2H]C1([C@@H]([C@@H]([C@@H](CO1)O)O)O)O |
Source
|
Source | PubChem | |
URL | https://pubchem.ncbi.nlm.nih.gov | |
Description | Data deposited in or computed by PubChem | |
Molecular Formula |
C5H10O5 |
Source
|
Source | PubChem | |
URL | https://pubchem.ncbi.nlm.nih.gov | |
Description | Data deposited in or computed by PubChem | |
Molecular Weight |
151.14 g/mol |
Source
|
Source | PubChem | |
URL | https://pubchem.ncbi.nlm.nih.gov | |
Description | Data deposited in or computed by PubChem | |
Disclaimer and Information on In-Vitro Research Products
Please be aware that all articles and product information presented on BenchChem are intended solely for informational purposes. The products available for purchase on BenchChem are specifically designed for in-vitro studies, which are conducted outside of living organisms. In-vitro studies, derived from the Latin term "in glass," involve experiments performed in controlled laboratory settings using cells or tissues. It is important to note that these products are not categorized as medicines or drugs, and they have not received approval from the FDA for the prevention, treatment, or cure of any medical condition, ailment, or disease. We must emphasize that any form of bodily introduction of these products into humans or animals is strictly prohibited by law. It is essential to adhere to these guidelines to ensure compliance with legal and ethical standards in research and experimentation.