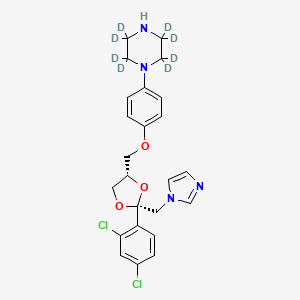
Deacetyl Ketoconazole-d8
- Click on QUICK INQUIRY to receive a quote from our team of experts.
- With the quality product at a COMPETITIVE price, you can focus more on your research.
Overview
Description
Deacetyl Ketoconazole-d8 is a derivative of Ketoconazole . Ketoconazole is an imidazole-containing antifungal drug . It has been used worldwide for 30 years, but its metabolic characteristics are poorly described . This compound is one of the metabolites of Ketoconazole .
Synthesis Analysis
Ketoconazole-d8 and N-deacetyl-ketoconazole were purchased from Toronto Research Chemicals . The metabolic characteristics of Ketoconazole, including reactive metabolites, were comprehensively understood using a metabolomics approach .Molecular Structure Analysis
The molecular structure of this compound involves a piperazine ring, imidazole ring, and N-acetyl moiety . The chemical formula is C24 H26 Cl2 N4 O3 .Chemical Reactions Analysis
A total of 28 metabolites of Ketoconazole, 11 of which were novel, were identified in a study . The oxidation of piperazine into iminium ion is suggested as a biotransformation responsible for bioactivation .Physical and Chemical Properties Analysis
The molecular weight of this compound is 489.39 . More detailed physical and chemical properties are not available in the retrieved papers.Scientific Research Applications
Characterization of Chemical-Induced Sterile Inflammation
Liver injury resulting from sterile inflammation is a significant concern in drug development. A study highlighted a novel in vitro co-culture model combining HepG2 and THP-1 cell lines to study the sterile inflammation caused by damaged hepatocytes. Ketoconazole was used as a model compound to test the co-culture. This model provided insights into the different metabolism patterns of ketoconazole and its main metabolite, N-deacetyl ketoconazole. The study emphasized the potential of this co-culture model as a tool for predicting chemical-induced sterile inflammation in liver tissue in vivo, showcasing the scientific research applications of ketoconazole and its metabolites in understanding drug-induced liver injury mechanisms (Wewering et al., 2017).
Drug-Drug Interaction Prediction Model
The scientific research application of ketoconazole also extends to predicting drug-drug interactions (DDIs) in humans. A novel approach was developed using cryopreserved human hepatocytes suspended in human plasma to mimic the in vivo concentration of ketoconazole at the enzymatic site. This study provided a method to predict DDIs for P450 substrates when co-administered with ketoconazole or other competitive P450 inhibitors. It demonstrated a simple, precise method for predicting DDIs, highlighting the significance of understanding the metabolic pathways and interactions of drugs like ketoconazole and its metabolites (Lu et al., 2007).
Mitochondrial Function Inhibition
Research has also explored the impact of ketoconazole and its metabolites, including deacetyl ketoconazole, on mitochondrial function. A study evaluating the metabolic integrity of adult rat liver mitochondria exposed to ketoconazole and its metabolites revealed dose-dependent inhibition of various metabolic pathways. The findings from this study are critical for understanding the toxicological aspects of drug metabolites and their impact on cellular metabolism, offering a scientific basis for assessing drug safety and toxicity (Rodriguez & Acosta, 1996).
Hepatocellular Toxicity and Enzyme Involvement
Investigations into the metabolism of ketoconazole and its correlation with hepatotoxicity identified human arylacetamide deacetylase (AADAC) as the enzyme responsible for ketoconazole hydrolysis to form deacetyl ketoconazole. This study highlighted the metabolic pathways of ketoconazole and its major metabolite, emphasizing their roles in drug-induced liver injury and the significance of enzymatic reactions in drug metabolism and toxicity (Fukami et al., 2016).
Mechanism of Action
Safety and Hazards
Future Directions
Despite the use of Ketoconazole worldwide for 30 years, its metabolic characteristics are poorly described . Future research could focus on further understanding the metabolic profile of Ketoconazole and its derivatives, including Deacetyl Ketoconazole-d8, and identifying the mechanisms underlying its hepatotoxicity .
Biochemical Analysis
Biochemical Properties
Deacetyl Ketoconazole-d8 plays a significant role in biochemical reactions, particularly in the study of drug metabolism and enzyme interactions. It interacts with several enzymes, including cytochrome P450 enzymes, which are crucial for the metabolism of many drugs. The interaction with these enzymes involves the inhibition of lanosterol 14α-demethylase, an enzyme essential for ergosterol biosynthesis in fungi . This inhibition disrupts the fungal cell membrane, leading to antifungal effects.
Cellular Effects
This compound affects various types of cells and cellular processes. In fungal cells, it inhibits the synthesis of ergosterol, a vital component of the cell membrane, leading to cell death. In mammalian cells, it can influence cell signaling pathways, gene expression, and cellular metabolism. For instance, it has been shown to induce the NRF2-mediated stress response and the CXCL8 (IL-8) pathway under co-culture conditions . These effects highlight its potential impact on cellular function and stress responses.
Molecular Mechanism
The molecular mechanism of this compound involves its binding interactions with biomolecules and enzyme inhibition. It binds to the heme iron of cytochrome P450 enzymes, inhibiting their activity. This inhibition prevents the conversion of lanosterol to ergosterol in fungi, disrupting cell membrane synthesis . Additionally, the compound’s deuterium labeling allows for detailed studies of its binding interactions and metabolic pathways.
Temporal Effects in Laboratory Settings
In laboratory settings, the effects of this compound can change over time. The compound’s stability and degradation are crucial factors in its long-term effects on cellular function. Studies have shown that the main metabolite, N-deacetyl ketoconazole, can be found in cell pellets but not in supernatants, indicating its accumulation within cells . This accumulation can lead to prolonged effects on cellular processes and potential toxicity.
Dosage Effects in Animal Models
The effects of this compound vary with different dosages in animal models. At lower doses, it effectively inhibits fungal growth without significant toxicity. At higher doses, it can cause hepatotoxicity and other adverse effects . These threshold effects highlight the importance of dosage optimization in therapeutic applications and research studies.
Metabolic Pathways
This compound is involved in several metabolic pathways, primarily through its interaction with cytochrome P450 enzymes. The compound undergoes extensive metabolism, resulting in various metabolites, including N-deacetyl ketoconazole . These metabolic pathways are crucial for understanding the compound’s pharmacokinetics and potential drug interactions.
Transport and Distribution
Within cells and tissues, this compound is transported and distributed through interactions with transporters and binding proteins. Its lipophilic nature allows it to accumulate in cell membranes, where it exerts its antifungal effects . The compound’s distribution is also influenced by its binding to plasma proteins, affecting its bioavailability and therapeutic efficacy.
Subcellular Localization
The subcellular localization of this compound is primarily within the cell membrane and cytoplasm. Its targeting signals and post-translational modifications direct it to specific compartments, where it interacts with enzymes and other biomolecules . This localization is essential for its activity and function in inhibiting fungal growth and affecting cellular processes.
Properties
IUPAC Name |
2,2,3,3,5,5,6,6-octadeuterio-1-[4-[[(2R,4S)-2-(2,4-dichlorophenyl)-2-(imidazol-1-ylmethyl)-1,3-dioxolan-4-yl]methoxy]phenyl]piperazine |
Source
|
---|---|---|
Source | PubChem | |
URL | https://pubchem.ncbi.nlm.nih.gov | |
Description | Data deposited in or computed by PubChem | |
InChI |
InChI=1S/C24H26Cl2N4O3/c25-18-1-6-22(23(26)13-18)24(16-29-10-7-28-17-29)32-15-21(33-24)14-31-20-4-2-19(3-5-20)30-11-8-27-9-12-30/h1-7,10,13,17,21,27H,8-9,11-12,14-16H2/t21-,24-/m0/s1/i8D2,9D2,11D2,12D2 |
Source
|
Source | PubChem | |
URL | https://pubchem.ncbi.nlm.nih.gov | |
Description | Data deposited in or computed by PubChem | |
InChI Key |
LOUXSEJZCPKWAX-LQQCIFTLSA-N |
Source
|
Source | PubChem | |
URL | https://pubchem.ncbi.nlm.nih.gov | |
Description | Data deposited in or computed by PubChem | |
Canonical SMILES |
C1CN(CCN1)C2=CC=C(C=C2)OCC3COC(O3)(CN4C=CN=C4)C5=C(C=C(C=C5)Cl)Cl |
Source
|
Source | PubChem | |
URL | https://pubchem.ncbi.nlm.nih.gov | |
Description | Data deposited in or computed by PubChem | |
Isomeric SMILES |
[2H]C1(C(N(C(C(N1)([2H])[2H])([2H])[2H])C2=CC=C(C=C2)OC[C@H]3CO[C@](O3)(CN4C=CN=C4)C5=C(C=C(C=C5)Cl)Cl)([2H])[2H])[2H] |
Source
|
Source | PubChem | |
URL | https://pubchem.ncbi.nlm.nih.gov | |
Description | Data deposited in or computed by PubChem | |
Molecular Formula |
C24H26Cl2N4O3 |
Source
|
Source | PubChem | |
URL | https://pubchem.ncbi.nlm.nih.gov | |
Description | Data deposited in or computed by PubChem | |
Molecular Weight |
497.4 g/mol |
Source
|
Source | PubChem | |
URL | https://pubchem.ncbi.nlm.nih.gov | |
Description | Data deposited in or computed by PubChem | |
Disclaimer and Information on In-Vitro Research Products
Please be aware that all articles and product information presented on BenchChem are intended solely for informational purposes. The products available for purchase on BenchChem are specifically designed for in-vitro studies, which are conducted outside of living organisms. In-vitro studies, derived from the Latin term "in glass," involve experiments performed in controlled laboratory settings using cells or tissues. It is important to note that these products are not categorized as medicines or drugs, and they have not received approval from the FDA for the prevention, treatment, or cure of any medical condition, ailment, or disease. We must emphasize that any form of bodily introduction of these products into humans or animals is strictly prohibited by law. It is essential to adhere to these guidelines to ensure compliance with legal and ethical standards in research and experimentation.