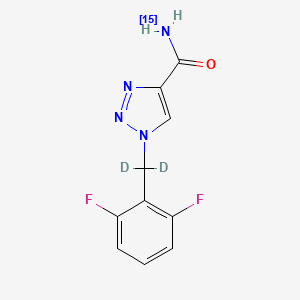
Rufinamide-15N,d2
Overview
Description
Rufinamide-15N-d2 is a deuterium and nitrogen-15 labeled derivative of Rufinamide, an anticonvulsant medication used primarily for the treatment of seizures associated with Lennox-Gastaut Syndrome. The labeling with deuterium and nitrogen-15 allows for precise tracking and quantification in metabolic studies, making it a valuable tool in scientific research .
Mechanism of Action
Target of Action
Rufinamide-15N,d2 is a deuterium and 15N labeled variant of Rufinamide . Rufinamide is an antiepileptic agent . The primary target of Rufinamide is the voltage-gated sodium channel 1.1 (Na v 1.1) . This channel plays a crucial role in the propagation of action potentials in neurons, and its dysfunction is associated with several neurological disorders, including epilepsy .
Mode of Action
This compound, like Rufinamide, acts by prolonging the inactive state of the Na v 1.1 channels . This action stabilizes the neuronal membranes, reducing the abnormal electrical activity in the brain that causes seizures . It’s important to note that Rufinamide selectively inhibits Na v 1.1, but not Na v 1.2, Na v 1.3, and Na v 1.6 .
Pharmacokinetics
This compound, like Rufinamide, is well absorbed with a bioavailability of at least 85% . Its volume of distribution is 0.71–1.14 L/kg, and plasma protein binding is 35% . Rufinamide is extensively metabolized in the liver, primarily by hydrolysis mediated by carboxylesterase 1 . Only about 2% of an administered dose is excreted as unchanged Rufinamide in urine . The plasma elimination half-life values for Rufinamide in adults are 6–10 hours .
Result of Action
The action of this compound results in the stabilization of neuronal membranes and a reduction in the abnormal electrical activity that causes seizures . This leads to a decrease in the frequency and severity of seizures in patients with Lennox-Gastaut syndrome (LGS), a form of childhood epilepsy .
Biochemical Analysis
Biochemical Properties
Rufinamide-15N,d2 plays a significant role in biochemical reactions, particularly in the context of its antiepileptic properties. It interacts with voltage-gated sodium channels, specifically inhibiting the activation of sodium channel 1.1 (Na v 1.1) at a concentration of 100 micromolar . This inhibition stabilizes the inactivated state of the sodium channels, thereby reducing neuronal excitability and preventing seizure activity. Additionally, this compound has been shown to inhibit carbonic anhydrase VA (CAVA) with a high degree of selectivity . This interaction further contributes to its anticonvulsant effects by modulating ion transport and pH regulation within neurons.
Cellular Effects
This compound exerts various effects on different types of cells and cellular processes. In primary rat hippocampal neurons, it increases the action potential threshold, thereby reducing neuronal excitability . This compound also prolongs the preictal phase and reduces the frequency of seizure-like events in an in vitro model of epileptiform activity . Furthermore, this compound has been shown to reduce kainic acid-induced neuronal cell death in the mouse hippocampal CA3 region, indicating its neuroprotective properties . These effects are mediated through the modulation of cell signaling pathways, gene expression, and cellular metabolism.
Molecular Mechanism
The molecular mechanism of this compound involves its interaction with voltage-gated sodium channels and carbonic anhydrase VA. By inhibiting the activation of sodium channel 1.1, this compound stabilizes the inactivated state of the channel, thereby reducing neuronal excitability . This inhibition is achieved through binding interactions with specific sites on the sodium channel protein. Additionally, this compound inhibits carbonic anhydrase VA, which plays a crucial role in ion transport and pH regulation within neurons . This dual mechanism of action contributes to its anticonvulsant and neuroprotective effects.
Temporal Effects in Laboratory Settings
In laboratory settings, the effects of this compound have been observed to change over time. The compound is relatively stable under standard storage conditions, with a shelf life of up to four years In in vitro studies, this compound has been shown to maintain its anticonvulsant properties over extended periods of exposure
Dosage Effects in Animal Models
The effects of this compound vary with different dosages in animal models. At lower doses, the compound effectively reduces seizure activity and provides neuroprotection without significant adverse effects . At higher doses, this compound may exhibit toxic effects, including potential hepatotoxicity and nephrotoxicity . These threshold effects highlight the importance of optimizing dosage regimens to maximize therapeutic benefits while minimizing adverse effects.
Metabolic Pathways
This compound is involved in several metabolic pathways, primarily through its interactions with enzymes such as carbonic anhydrase VA . The compound undergoes hydrolysis by carboxylesterases to form a pharmacologically inactive carboxylic acid derivative, which is subsequently excreted in the urine . This metabolic pathway ensures the efficient clearance of this compound from the body, thereby reducing the risk of accumulation and toxicity.
Transport and Distribution
Within cells and tissues, this compound is transported and distributed through interactions with specific transporters and binding proteins. The compound is not extensively bound to plasma proteins, allowing for efficient distribution to target tissues . In neurons, this compound accumulates in regions with high sodium channel density, such as the hippocampus and cortex . This targeted distribution enhances its anticonvulsant and neuroprotective effects.
Subcellular Localization
This compound exhibits specific subcellular localization, which influences its activity and function. The compound is primarily localized to the plasma membrane and cytoplasm of neurons, where it interacts with voltage-gated sodium channels and carbonic anhydrase VA . This localization is facilitated by targeting signals and post-translational modifications that direct this compound to specific compartments within the cell. The precise subcellular distribution of this compound is crucial for its therapeutic efficacy and safety.
Preparation Methods
Synthetic Routes and Reaction Conditions: The synthesis of Rufinamide-15N-d2 involves the incorporation of deuterium and nitrogen-15 into the Rufinamide molecule. The process typically starts with the preparation of the labeled starting materials, followed by a series of chemical reactions to introduce the labeled atoms into the desired positions within the molecule. Common reagents used in these reactions include deuterated solvents and nitrogen-15 labeled ammonia .
Industrial Production Methods: Industrial production of Rufinamide-15N-d2 follows similar synthetic routes but on a larger scale. The process involves stringent quality control measures to ensure the purity and consistency of the labeled compound. Techniques such as gas chromatography and liquid chromatography are employed to monitor the synthesis and confirm the incorporation of the labeled atoms .
Chemical Reactions Analysis
Types of Reactions: Rufinamide-15N-d2 undergoes various chemical reactions, including:
Reduction: Involves the removal of oxygen atoms or the addition of hydrogen atoms.
Substitution: Involves the replacement of one atom or group of atoms with another.
Common Reagents and Conditions:
Oxidation: Common reagents include hydrogen peroxide and potassium permanganate.
Reduction: Common reagents include sodium borohydride and lithium aluminum hydride.
Substitution: Common reagents include halogens and nucleophiles such as hydroxide ions
Major Products: The major products formed from these reactions depend on the specific conditions and reagents used. For example, oxidation may yield hydroxylated derivatives, while reduction may yield deuterated analogs .
Scientific Research Applications
Rufinamide-15N-d2 has a wide range of applications in scientific research, including:
Chemistry: Used as an internal standard for the quantification of Rufinamide in analytical studies.
Biology: Employed in metabolic studies to track the distribution and transformation of Rufinamide in biological systems.
Medicine: Utilized in pharmacokinetic studies to understand the absorption, distribution, metabolism, and excretion of Rufinamide.
Industry: Applied in the development of new anticonvulsant medications and in quality control processes .
Comparison with Similar Compounds
Rufinamide: The parent compound, used as an anticonvulsant.
Rufinamide-d2: A deuterium-labeled derivative without nitrogen-15 labeling.
Rufinamide-15N: A nitrogen-15 labeled derivative without deuterium labeling
Uniqueness: Rufinamide-15N-d2 is unique due to its dual labeling with both deuterium and nitrogen-15, allowing for more precise tracking and quantification in metabolic studies compared to its singly labeled counterparts .
Properties
IUPAC Name |
1-[dideuterio-(2,6-difluorophenyl)methyl]triazole-4-(15N)carboxamide | |
---|---|---|
Source | PubChem | |
URL | https://pubchem.ncbi.nlm.nih.gov | |
Description | Data deposited in or computed by PubChem | |
InChI |
InChI=1S/C10H8F2N4O/c11-7-2-1-3-8(12)6(7)4-16-5-9(10(13)17)14-15-16/h1-3,5H,4H2,(H2,13,17)/i4D2,13+1 | |
Source | PubChem | |
URL | https://pubchem.ncbi.nlm.nih.gov | |
Description | Data deposited in or computed by PubChem | |
InChI Key |
POGQSBRIGCQNEG-RPVANBMVSA-N | |
Source | PubChem | |
URL | https://pubchem.ncbi.nlm.nih.gov | |
Description | Data deposited in or computed by PubChem | |
Canonical SMILES |
C1=CC(=C(C(=C1)F)CN2C=C(N=N2)C(=O)N)F | |
Source | PubChem | |
URL | https://pubchem.ncbi.nlm.nih.gov | |
Description | Data deposited in or computed by PubChem | |
Isomeric SMILES |
[2H]C([2H])(C1=C(C=CC=C1F)F)N2C=C(N=N2)C(=O)[15NH2] | |
Source | PubChem | |
URL | https://pubchem.ncbi.nlm.nih.gov | |
Description | Data deposited in or computed by PubChem | |
Molecular Formula |
C10H8F2N4O | |
Source | PubChem | |
URL | https://pubchem.ncbi.nlm.nih.gov | |
Description | Data deposited in or computed by PubChem | |
Molecular Weight |
241.20 g/mol | |
Source | PubChem | |
URL | https://pubchem.ncbi.nlm.nih.gov | |
Description | Data deposited in or computed by PubChem | |
Synthesis routes and methods I
Procedure details
Synthesis routes and methods II
Procedure details
Synthesis routes and methods III
Procedure details
Synthesis routes and methods IV
Procedure details
Synthesis routes and methods V
Procedure details
Retrosynthesis Analysis
AI-Powered Synthesis Planning: Our tool employs the Template_relevance Pistachio, Template_relevance Bkms_metabolic, Template_relevance Pistachio_ringbreaker, Template_relevance Reaxys, Template_relevance Reaxys_biocatalysis model, leveraging a vast database of chemical reactions to predict feasible synthetic routes.
One-Step Synthesis Focus: Specifically designed for one-step synthesis, it provides concise and direct routes for your target compounds, streamlining the synthesis process.
Accurate Predictions: Utilizing the extensive PISTACHIO, BKMS_METABOLIC, PISTACHIO_RINGBREAKER, REAXYS, REAXYS_BIOCATALYSIS database, our tool offers high-accuracy predictions, reflecting the latest in chemical research and data.
Strategy Settings
Precursor scoring | Relevance Heuristic |
---|---|
Min. plausibility | 0.01 |
Model | Template_relevance |
Template Set | Pistachio/Bkms_metabolic/Pistachio_ringbreaker/Reaxys/Reaxys_biocatalysis |
Top-N result to add to graph | 6 |
Feasible Synthetic Routes
Disclaimer and Information on In-Vitro Research Products
Please be aware that all articles and product information presented on BenchChem are intended solely for informational purposes. The products available for purchase on BenchChem are specifically designed for in-vitro studies, which are conducted outside of living organisms. In-vitro studies, derived from the Latin term "in glass," involve experiments performed in controlled laboratory settings using cells or tissues. It is important to note that these products are not categorized as medicines or drugs, and they have not received approval from the FDA for the prevention, treatment, or cure of any medical condition, ailment, or disease. We must emphasize that any form of bodily introduction of these products into humans or animals is strictly prohibited by law. It is essential to adhere to these guidelines to ensure compliance with legal and ethical standards in research and experimentation.