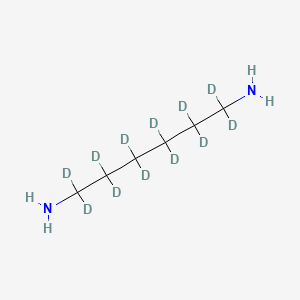
1,6-Diaminohexane-d12
- Click on QUICK INQUIRY to receive a quote from our team of experts.
- With the quality product at a COMPETITIVE price, you can focus more on your research.
Overview
Description
It is a linear aliphatic diamine with the molecular formula H2NCD2(CD2)4CD2NH2 and a molecular weight of 128.28 g/mol . This compound is characterized by the presence of deuterium atoms, which replace the hydrogen atoms in the non-deuterated form. The deuterium labeling makes it particularly useful in various scientific research applications, including nuclear magnetic resonance (NMR) spectroscopy.
Preparation Methods
Synthetic Routes and Reaction Conditions
1,6-Diaminohexane-d12 can be synthesized through the catalytic hydrogenation of adiponitrile-d12. The reaction involves the use of a deuterium gas atmosphere and a suitable catalyst, such as Raney nickel or palladium on carbon, under high pressure and temperature conditions . The reaction proceeds as follows:
NC-(CD2)4-CN+4D2→H2N-(CD2)6-NH2
Industrial Production Methods
Industrial production of this compound typically involves the same catalytic hydrogenation process but on a larger scale. The process requires stringent control of reaction conditions to ensure high yield and purity of the deuterated product. The use of high-purity deuterium gas and advanced catalytic systems is essential for efficient production .
Chemical Reactions Analysis
Types of Reactions
1,6-Diaminohexane-d12 undergoes various chemical reactions, including:
Oxidation: It can be oxidized to form corresponding amides or nitriles.
Reduction: It can be reduced to form primary amines.
Substitution: It can participate in nucleophilic substitution reactions to form various derivatives.
Common Reagents and Conditions
Oxidation: Common oxidizing agents include potassium permanganate and hydrogen peroxide.
Reduction: Reducing agents such as lithium aluminum hydride or sodium borohydride are used.
Substitution: Reagents like alkyl halides or acyl chlorides are commonly used in substitution reactions.
Major Products Formed
Oxidation: Formation of hexanediamide or hexanedinitrile.
Reduction: Formation of hexylamine.
Substitution: Formation of N-alkyl or N-acyl derivatives.
Scientific Research Applications
1,6-Diaminohexane-d12 is widely used in scientific research due to its deuterium labeling. Some of its applications include:
NMR Spectroscopy: The deuterium atoms provide a distinct signal in NMR spectra, making it useful for studying molecular structures and dynamics.
Isotope Labeling: It is used as an isotope-labeled standard in mass spectrometry and other analytical techniques.
Polymer Chemistry: It is used in the synthesis of deuterated polymers for studying polymerization mechanisms and properties
Mechanism of Action
The mechanism of action of 1,6-diaminohexane-d12 is primarily related to its role as a diamine. It can interact with various molecular targets, including enzymes and receptors, through hydrogen bonding and electrostatic interactions. The deuterium atoms can influence the kinetic isotope effect, altering reaction rates and pathways. This makes it a valuable tool for studying reaction mechanisms and enzyme kinetics .
Comparison with Similar Compounds
Similar Compounds
1,6-Diaminohexane: The non-deuterated form, commonly used in the production of nylon-6,6.
1,6-Diaminohexane-3,3,4,4-d4: A partially deuterated form with deuterium atoms at specific positions.
1,6-Diaminohexane-1,1,6,6-d4: Another partially deuterated form with deuterium atoms at different positions.
Uniqueness
1,6-Diaminohexane-d12 is unique due to its complete deuteration, which provides distinct advantages in NMR spectroscopy and other analytical techniques. The presence of deuterium atoms enhances the resolution and sensitivity of analytical methods, making it a preferred choice for detailed molecular studies .
Biological Activity
1,6-Diaminohexane-d12, also known as hexamethylenediamine-d12, is a deuterated form of 1,6-diaminohexane. This compound has garnered attention for its potential biological activities, particularly in the fields of pharmacology and toxicology. This article provides a comprehensive overview of its biological activity based on available research findings.
This compound has the molecular formula C6H16N2 and is characterized by two amine groups at either end of a six-carbon alkane chain. The presence of deuterium isotopes enhances its stability and makes it useful in various analytical applications.
Toxicological Profile
The toxicological profile of this compound is closely related to its non-deuterated counterpart, 1,6-hexanediamine. Studies have shown that:
- Acute Toxicity : The oral LD50 (lethal dose for 50% of the population) for 1,6-hexanediamine is approximately 980 mg/kg in rats . Similar toxicity levels are expected for the deuterated variant due to its structural similarity.
- Chronic Exposure : In long-term studies, administration at doses of 0, 50, 150, and 500 mg/kg resulted in modest weight gain retardation at higher doses without significant organ toxicity or changes in blood parameters .
Metabolism and Excretion
Research indicates that following administration, a significant portion of the compound is excreted unchanged. For example:
- In a study involving rats, about 27% of the administered dose was found in feces, while 20% was exhaled as carbon dioxide .
- The highest specific activity was noted in the prostate and intestines shortly after intravenous administration .
Study on Antibacterial Activity
A notable study explored the antibacterial properties of Schiff bases derived from 1,6-diaminohexane. The research highlighted:
- Synthesis : Schiff bases were synthesized using various aldehydes and characterized using spectroscopic methods.
- Biological Testing : These derivatives exhibited significant antibacterial activity against several strains of bacteria. The study employed quantitative structure-activity relationship (QSAR) analysis to correlate chemical structure with biological efficacy .
Environmental Applications
This compound has also been utilized in environmental studies to trace chemical movements and behaviors in various ecosystems. Its isotopic labeling allows for precise tracking in complex matrices .
Data Summary Table
Parameter | Value |
---|---|
Molecular Formula | C₆H₁₆N₂ |
Oral LD50 | ~980 mg/kg (rats) |
Metabolism | Excreted as unchanged compound |
Antibacterial Activity | Significant against various bacteria |
Environmental Tracking | Effective due to isotopic labeling |
Q & A
Basic Research Questions
Q. What are the standard synthesis protocols for 1,6-Diaminohexane-d12 in polymer chemistry applications?
- Methodological Answer : Synthesis typically involves deuterating 1,6-diaminohexane (HMDA) via catalytic exchange in deuterated solvents (e.g., D₂O or DCl). Characterization requires nuclear magnetic resonance (NMR) and Fourier-transform infrared spectroscopy (FTIR) to confirm deuteration efficiency (>98%) and amine group integrity. Refer to copolymerization protocols in polyimide foams, where HMDA-d12 is reacted with aromatic dianhydrides under controlled humidity to prevent hygroscopic interference .
Q. Which analytical techniques are critical for characterizing this compound in epoxy curing systems?
- Methodological Answer : Differential scanning calorimetry (DSC) monitors curing exotherms and glass transition temperatures (Tg). Dynamic mechanical analysis (DMA) evaluates crosslink density and network formation. For kinetic studies, use in-situ FTIR to track amine-epoxy reactions and gelation points. Ensure solvent-free conditions to avoid side reactions with hygroscopic HMDA-d12 .
Q. How should researchers handle the hygroscopic nature of this compound during experimental preparation?
- Methodological Answer : Store HMDA-d12 in desiccators with anhydrous calcium sulfate. Conduct reactions under inert gas (N₂/Ar) to minimize moisture absorption. Pre-dry solvents (e.g., tetrahydrofuran) over molecular sieves. Gravimetric analysis pre- and post-experiment can quantify moisture uptake, which may alter reaction stoichiometry .
Q. What is the role of this compound in modifying polyimide foam morphology?
- Methodological Answer : Incorporating HMDA-d12 into aromatic polyimides introduces flexible aliphatic segments, reducing rigidity and increasing open-cell ratios. Optimize molar ratios (e.g., 10–20 mol%) via step-growth polymerization. Scanning electron microscopy (SEM) reveals larger cell diameters and lowered density compared to purely aromatic systems .
Advanced Research Questions
Q. How can autocatalytic curing kinetics of HMDA-d12-epoxy systems be modeled for industrial adhesives?
- Methodological Answer : Apply the extended Kamal model to isothermal DSC data, incorporating diffusion-controlled terms post-vitrification. Fit rate constants (k₁, k₂) and activation energy (Eₐ) using the Málek method. For HMDA-d12, compare kinetic parameters with non-deuterated analogs to assess isotope effects on reactivity .
Q. What strategies resolve contradictions in thermal stability data for HMDA-d12-modified copolymers?
- Methodological Answer : Contradictions often arise from varying deuteration levels or residual solvents. Use thermogravimetric analysis (TGA) coupled with mass spectrometry (TGA-MS) to differentiate decomposition pathways. Cross-validate with X-ray photoelectron spectroscopy (XPS) to quantify surface vs. bulk deuterium content .
Q. How does copolymer architecture influence the mechanical properties of HMDA-d12-based polyimides?
- Methodological Answer : Tailor copolymer sequences via controlled monomer addition (e.g., block vs. random). Tensile testing and DMA reveal trade-offs: higher HMDA-d12 content reduces Young’s modulus but enhances strain-at-break. Molecular dynamics simulations predict segmental mobility, validated by positron annihilation lifetime spectroscopy (PALS) .
Q. What experimental designs validate the isotopic labeling efficiency of HMDA-d12 in metabolic tracer studies?
- Methodological Answer : Use high-resolution mass spectrometry (HRMS) and ²H-NMR to quantify isotopic purity. In cell cultures, track deuterium incorporation into biomarkers via liquid chromatography-tandem MS (LC-MS/MS). Control for metabolic scrambling by comparing with ¹³C-labeled analogs .
Q. How does deuteration impact the nucleophilicity of HMDA-d12 in epoxy-amine reactions?
- Methodological Answer : Kinetic isotope effects (KIE) reduce reaction rates due to stronger N-D bonds. Conduct competitive reactions between HMDA and HMDA-d12 with excess epoxy. Monitor via ¹H/²H NMR integration. For DGEBA systems, expect KIE values of 2–3, altering gelation times .
Q. What advanced techniques analyze network heterogeneity in HMDA-d12-cured epoxy resins?
Properties
IUPAC Name |
1,1,2,2,3,3,4,4,5,5,6,6-dodecadeuteriohexane-1,6-diamine |
Source
|
---|---|---|
Source | PubChem | |
URL | https://pubchem.ncbi.nlm.nih.gov | |
Description | Data deposited in or computed by PubChem | |
InChI |
InChI=1S/C6H16N2/c7-5-3-1-2-4-6-8/h1-8H2/i1D2,2D2,3D2,4D2,5D2,6D2 |
Source
|
Source | PubChem | |
URL | https://pubchem.ncbi.nlm.nih.gov | |
Description | Data deposited in or computed by PubChem | |
InChI Key |
NAQMVNRVTILPCV-LBTWDOQPSA-N |
Source
|
Source | PubChem | |
URL | https://pubchem.ncbi.nlm.nih.gov | |
Description | Data deposited in or computed by PubChem | |
Canonical SMILES |
C(CCCN)CCN |
Source
|
Source | PubChem | |
URL | https://pubchem.ncbi.nlm.nih.gov | |
Description | Data deposited in or computed by PubChem | |
Isomeric SMILES |
[2H]C([2H])(C([2H])([2H])C([2H])([2H])C([2H])([2H])N)C([2H])([2H])C([2H])([2H])N |
Source
|
Source | PubChem | |
URL | https://pubchem.ncbi.nlm.nih.gov | |
Description | Data deposited in or computed by PubChem | |
Molecular Formula |
C6H16N2 |
Source
|
Source | PubChem | |
URL | https://pubchem.ncbi.nlm.nih.gov | |
Description | Data deposited in or computed by PubChem | |
Molecular Weight |
128.28 g/mol |
Source
|
Source | PubChem | |
URL | https://pubchem.ncbi.nlm.nih.gov | |
Description | Data deposited in or computed by PubChem | |
Disclaimer and Information on In-Vitro Research Products
Please be aware that all articles and product information presented on BenchChem are intended solely for informational purposes. The products available for purchase on BenchChem are specifically designed for in-vitro studies, which are conducted outside of living organisms. In-vitro studies, derived from the Latin term "in glass," involve experiments performed in controlled laboratory settings using cells or tissues. It is important to note that these products are not categorized as medicines or drugs, and they have not received approval from the FDA for the prevention, treatment, or cure of any medical condition, ailment, or disease. We must emphasize that any form of bodily introduction of these products into humans or animals is strictly prohibited by law. It is essential to adhere to these guidelines to ensure compliance with legal and ethical standards in research and experimentation.