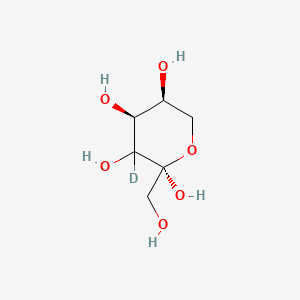
D-Fructose-3-d
- Click on QUICK INQUIRY to receive a quote from our team of experts.
- With the quality product at a COMPETITIVE price, you can focus more on your research.
Overview
Description
D-Fructose-3-d, also known as D-allulose, is a rare sugar that is a C-3 epimer of D-fructose. It is naturally present in small quantities in various foods and has gained attention due to its potential health benefits and applications in the food industry. Unlike regular sugars, D-allulose has a low caloric value and does not significantly raise blood glucose levels, making it an attractive alternative sweetener.
Mechanism of Action
Target of Action
D-Fructose-3-d, also known as D-allulose, primarily targets enzymes such as D-allulose 3-epimerase (DAEase) or D-psicose 3-epimerase (DPEase) to convert D-fructose into D-allulose . These enzymes play a crucial role in the isomerization process of D-fructose .
Mode of Action
The mode of action of this compound involves the isomerization of D-fructose to D-allulose. This process is catalyzed by the enzymes DAEase or DPEase . The catalytic reaction of these enzymes occurs in three major steps: ring opening, isomerization, and ring closure .
Biochemical Pathways
This compound affects the sugar metabolism pathway. It is involved in the isomerization of D-fructose to D-allulose, a process that is part of the broader carbohydrate metabolism . This process is crucial in fulfilling nutritional requirements in bacteria . Additionally, this compound is also involved in the production of high-fructose corn syrup and bioethanol .
Pharmacokinetics
This compound exhibits high metabolic stability. It is neither pH-labile nor degraded in the gastrointestinal tract, indicating high bioavailability .
Result of Action
The result of this compound action is the production of D-allulose from D-fructose. D-allulose is a rare sugar with unique nutritional and biological functions . It has been found to have potential health benefits, making it a valuable compound in the food, pharmaceutical, and medical industries .
Action Environment
The action of this compound can be influenced by environmental factors. For instance, the efficiency of the isomerization process can be affected by the thermotolerance of the enzymes involved . Research advancements in the engineering of these enzymes for improved thermotolerance or acid resistance have been made to enhance the yield of D-allulose .
Preparation Methods
Synthetic Routes and Reaction Conditions: D-Fructose-3-d can be synthesized from D-fructose through an enzymatic epimerization process. The key enzyme used in this process is D-allulose 3-epimerase, which catalyzes the conversion of D-fructose to D-allulose. The reaction typically occurs under mild conditions, with optimal temperatures ranging from 50°C to 60°C and a neutral to slightly acidic pH .
Industrial Production Methods: Industrial production of D-allulose involves the use of microbial fermentation and enzymatic conversion. Microorganisms such as Bacillus subtilis and Escherichia coli are genetically engineered to express D-allulose 3-epimerase, enabling efficient conversion of D-fructose to D-allulose. The process is optimized to achieve high yields and purity, with minimal by-products .
Chemical Reactions Analysis
Types of Reactions: D-Fructose-3-d undergoes various chemical reactions, including:
Reduction: Reduction of D-allulose can yield sugar alcohols such as D-allitol.
Isomerization: D-allulose can be isomerized back to D-fructose under specific conditions.
Common Reagents and Conditions:
Reduction: Metal catalysts like palladium on carbon (Pd/C) are used for the reduction of D-allulose to sugar alcohols.
Isomerization: Base catalysts such as sodium hydroxide (NaOH) facilitate the isomerization of D-allulose to D-fructose.
Major Products:
5-Hydroxymethylfurfural (HMF): Produced from the oxidation of D-allulose.
D-Allitol: Produced from the reduction of D-allulose.
Scientific Research Applications
D-Fructose-3-d has a wide range of applications in scientific research:
Chemistry: Used as a precursor for the synthesis of various chemicals and materials.
Comparison with Similar Compounds
D-Glucose: A common hexose sugar with a similar structure but different metabolic effects.
D-Fructose: The parent compound of D-allulose, with higher caloric value and greater impact on blood glucose levels.
D-Tagatose: Another rare sugar with similar health benefits but different enzymatic pathways for its production.
Uniqueness of D-Fructose-3-d: this compound stands out due to its low caloric value, minimal impact on blood glucose levels, and potential health benefits. Its unique enzymatic production process and diverse applications in food, medicine, and industry further highlight its significance.
Properties
IUPAC Name |
(2S,4S,5S)-3-deuterio-2-(hydroxymethyl)oxane-2,3,4,5-tetrol |
Source
|
---|---|---|
Source | PubChem | |
URL | https://pubchem.ncbi.nlm.nih.gov | |
Description | Data deposited in or computed by PubChem | |
InChI |
InChI=1S/C6H12O6/c7-2-6(11)5(10)4(9)3(8)1-12-6/h3-5,7-11H,1-2H2/t3-,4-,5?,6-/m0/s1/i5D |
Source
|
Source | PubChem | |
URL | https://pubchem.ncbi.nlm.nih.gov | |
Description | Data deposited in or computed by PubChem | |
InChI Key |
LKDRXBCSQODPBY-BUZGMKGYSA-N |
Source
|
Source | PubChem | |
URL | https://pubchem.ncbi.nlm.nih.gov | |
Description | Data deposited in or computed by PubChem | |
Canonical SMILES |
C1C(C(C(C(O1)(CO)O)O)O)O |
Source
|
Source | PubChem | |
URL | https://pubchem.ncbi.nlm.nih.gov | |
Description | Data deposited in or computed by PubChem | |
Isomeric SMILES |
[2H]C1([C@H]([C@H](CO[C@]1(CO)O)O)O)O |
Source
|
Source | PubChem | |
URL | https://pubchem.ncbi.nlm.nih.gov | |
Description | Data deposited in or computed by PubChem | |
Molecular Formula |
C6H12O6 |
Source
|
Source | PubChem | |
URL | https://pubchem.ncbi.nlm.nih.gov | |
Description | Data deposited in or computed by PubChem | |
Molecular Weight |
181.16 g/mol |
Source
|
Source | PubChem | |
URL | https://pubchem.ncbi.nlm.nih.gov | |
Description | Data deposited in or computed by PubChem | |
Q1: What information can be obtained from the 13C NMR spectrum of D-Fructose-3-d regarding its structure and behavior in solution?
A1: The research article "Conformational analysis in carbohydrate chemistry. III. The 13C N.M.R. spectra of the hexuloses" [] investigates the use of 13C NMR spectroscopy to analyze the structure and behavior of hexuloses, including D-fructose, in solution. While the study doesn't specifically focus on this compound, it provides valuable insights applicable to this deuterated derivative.
- Analyze conformational equilibrium: The study demonstrates that the 13C NMR spectrum reflects the equilibrium between different conformations of hexuloses in solution []. Analyzing the chemical shifts and relative intensities of peaks corresponding to different conformers of this compound can provide insights into how deuterium substitution influences the conformational preferences of the molecule.
Disclaimer and Information on In-Vitro Research Products
Please be aware that all articles and product information presented on BenchChem are intended solely for informational purposes. The products available for purchase on BenchChem are specifically designed for in-vitro studies, which are conducted outside of living organisms. In-vitro studies, derived from the Latin term "in glass," involve experiments performed in controlled laboratory settings using cells or tissues. It is important to note that these products are not categorized as medicines or drugs, and they have not received approval from the FDA for the prevention, treatment, or cure of any medical condition, ailment, or disease. We must emphasize that any form of bodily introduction of these products into humans or animals is strictly prohibited by law. It is essential to adhere to these guidelines to ensure compliance with legal and ethical standards in research and experimentation.