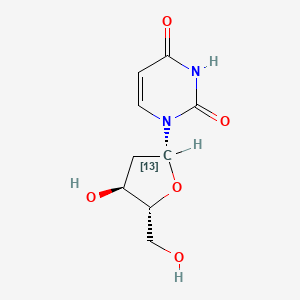
2'-Deoxyuridine-1 inverted exclamation marka-13C
- Click on QUICK INQUIRY to receive a quote from our team of experts.
- With the quality product at a COMPETITIVE price, you can focus more on your research.
Overview
Description
[1’-13C]2’-Deoxyuridine: is a labeled nucleoside analog where the carbon-13 isotope is incorporated at the 1’ position of the deoxyribose sugar. This compound is a derivative of 2’-deoxyuridine, a pyrimidine nucleoside that plays a crucial role in DNA synthesis. The incorporation of the carbon-13 isotope makes it particularly useful in various scientific research applications, including metabolic studies and tracing experiments.
Mechanism of Action
Target of Action
The primary targets of [1’-13C]2’-Deoxyuridine are Uridine Phosphorylase and Thymidylate Synthase . These enzymes play a crucial role in the metabolism of nucleosides, which are essential components of DNA and RNA.
Mode of Action
[1’-13C]2’-Deoxyuridine is an antimetabolite that gets converted to deoxyuridine triphosphate during DNA synthesis . It interacts with its targets by substituting itself for thymidine in the DNA structure, thereby inhibiting the proper functioning of thymidylate phosphorylase and viral DNA polymerases .
Biochemical Pathways
The compound affects the biochemical pathways involved in DNA synthesis and repair. It is incorporated into the DNA structure during replication, leading to the production of faulty DNA . This process involves the recognition and removal of damaged or wrong bases by uracil DNA glycosylase .
Pharmacokinetics
It is known that the compound is converted to deoxyuridine triphosphate during dna synthesis
Result of Action
The result of [1’-13C]2’-Deoxyuridine’s action is the production of faulty DNA, which cannot infect or destroy tissue . This is due to the compound’s ability to replace thymidine in the DNA structure, thereby inhibiting the proper functioning of thymidylate phosphorylase and viral DNA polymerases .
Biochemical Analysis
Biochemical Properties
[1’-13C]2’-Deoxyuridine interacts with various enzymes and proteins during biochemical reactions. It is converted to deoxyuridine triphosphate during DNA synthesis . This conversion is essential for the formation of DNA, indicating the significant role of [1’-13C]2’-Deoxyuridine in DNA replication and cell division .
Cellular Effects
The effects of [1’-13C]2’-Deoxyuridine on cells are profound. It has been found to enhance the growth of Chinese hamster ovary (CHO) cells, leading to an increase in antibody concentration . Additionally, [1’-13C]2’-Deoxyuridine has been observed to have an inhibitory and promutagenic effect on RNA transcription .
Molecular Mechanism
The molecular mechanism of [1’-13C]2’-Deoxyuridine involves its conversion to deoxyuridine triphosphate during DNA synthesis . This process is crucial for the formation of DNA, indicating the significant role of [1’-13C]2’-Deoxyuridine at the molecular level .
Temporal Effects in Laboratory Settings
The effects of [1’-13C]2’-Deoxyuridine can change over time in laboratory settings. For instance, it has been observed to have an inhibitory and promutagenic effect on RNA transcription
Metabolic Pathways
[1’-13C]2’-Deoxyuridine is involved in the metabolic pathway of DNA synthesis, where it is converted to deoxyuridine triphosphate . This process involves various enzymes and cofactors, and is crucial for the formation of DNA .
Preparation Methods
Synthetic Routes and Reaction Conditions: The synthesis of [1’-13C]2’-Deoxyuridine typically involves the incorporation of the carbon-13 isotope into the deoxyribose sugar, followed by the coupling of this labeled sugar with uracil. The process can be summarized as follows:
Synthesis of [1’-13C]Deoxyribose: The labeled deoxyribose is synthesized using a carbon-13 labeled precursor, such as [13C]formaldehyde, through a series of chemical reactions including reduction and protection steps.
Coupling with Uracil: The labeled deoxyribose is then coupled with uracil using a glycosylation reaction. This step typically involves the use of a Lewis acid catalyst, such as trimethylsilyl trifluoromethanesulfonate, under anhydrous conditions.
Industrial Production Methods: Industrial production of [1’-13C]2’-Deoxyuridine follows similar synthetic routes but on a larger scale. The process involves:
Bulk Synthesis of [1’-13C]Deoxyribose: Large-scale synthesis of the labeled deoxyribose using optimized reaction conditions to ensure high yield and purity.
Automated Glycosylation: The coupling reaction is often automated to increase efficiency and consistency. Advanced purification techniques, such as high-performance liquid chromatography, are used to isolate the final product.
Chemical Reactions Analysis
Types of Reactions:
Oxidation: [1’-13C]2’-Deoxyuridine can undergo oxidation reactions, particularly at the 5-position of the uracil ring, leading to the formation of 5-hydroxy-2’-deoxyuridine.
Reduction: Reduction reactions can convert the uracil ring to dihydrouracil derivatives.
Substitution: Halogenation reactions can introduce halogen atoms at the 5-position, resulting in compounds like 5-bromo-2’-deoxyuridine.
Common Reagents and Conditions:
Oxidation: Hydrogen peroxide or potassium permanganate under acidic conditions.
Reduction: Sodium borohydride or catalytic hydrogenation.
Substitution: N-bromosuccinimide for bromination, often in the presence of a radical initiator like azobisisobutyronitrile.
Major Products:
Oxidation: 5-Hydroxy-2’-deoxyuridine.
Reduction: Dihydrouracil derivatives.
Substitution: 5-Bromo-2’-deoxyuridine.
Scientific Research Applications
Chemistry:
Isotope Labeling: [1’-13C]2’-Deoxyuridine is used as a tracer in metabolic studies to track the incorporation and turnover of nucleosides in DNA synthesis.
Structural Studies: The carbon-13 label allows for detailed nuclear magnetic resonance (NMR) spectroscopy studies to elucidate the structure and dynamics of nucleic acids.
Biology:
DNA Synthesis Studies: It is used to investigate the mechanisms of DNA replication and repair by incorporating the labeled nucleoside into DNA strands.
Cell Cycle Analysis: The compound is used in cell proliferation assays to monitor DNA synthesis during different phases of the cell cycle.
Medicine:
Cancer Research: [1’-13C]2’-Deoxyuridine is used in studies to understand the metabolism of cancer cells and to develop targeted therapies.
Diagnostic Imaging: The labeled compound can be used in positron emission tomography (PET) imaging to study cellular proliferation in tumors.
Industry:
Pharmaceutical Development: It is used in the development of new nucleoside analog drugs by providing insights into their metabolic pathways and mechanisms of action.
Comparison with Similar Compounds
2’-Deoxyuridine: The unlabeled parent compound used in similar applications but without the isotope labeling.
5-Bromo-2’-Deoxyuridine: A halogenated derivative used in DNA labeling and cancer research.
5-Iodo-2’-Deoxyuridine: Another halogenated derivative with antiviral and anticancer properties.
Uniqueness:
Isotope Labeling: The incorporation of the carbon-13 isotope makes [1’-13C]2’-Deoxyuridine unique for tracing and metabolic studies.
Detection Sensitivity: The carbon-13 label enhances the sensitivity and specificity of detection methods like NMR and mass spectrometry, providing more detailed insights into nucleoside metabolism and DNA synthesis.
Properties
IUPAC Name |
1-[(2R,4S,5R)-4-hydroxy-5-(hydroxymethyl)(213C)oxolan-2-yl]pyrimidine-2,4-dione |
Source
|
---|---|---|
Source | PubChem | |
URL | https://pubchem.ncbi.nlm.nih.gov | |
Description | Data deposited in or computed by PubChem | |
InChI |
InChI=1S/C9H12N2O5/c12-4-6-5(13)3-8(16-6)11-2-1-7(14)10-9(11)15/h1-2,5-6,8,12-13H,3-4H2,(H,10,14,15)/t5-,6+,8+/m0/s1/i8+1 |
Source
|
Source | PubChem | |
URL | https://pubchem.ncbi.nlm.nih.gov | |
Description | Data deposited in or computed by PubChem | |
InChI Key |
MXHRCPNRJAMMIM-ROGMPORQSA-N |
Source
|
Source | PubChem | |
URL | https://pubchem.ncbi.nlm.nih.gov | |
Description | Data deposited in or computed by PubChem | |
Canonical SMILES |
C1C(C(OC1N2C=CC(=O)NC2=O)CO)O |
Source
|
Source | PubChem | |
URL | https://pubchem.ncbi.nlm.nih.gov | |
Description | Data deposited in or computed by PubChem | |
Isomeric SMILES |
C1[C@@H]([C@H](O[13C@H]1N2C=CC(=O)NC2=O)CO)O |
Source
|
Source | PubChem | |
URL | https://pubchem.ncbi.nlm.nih.gov | |
Description | Data deposited in or computed by PubChem | |
Molecular Formula |
C9H12N2O5 |
Source
|
Source | PubChem | |
URL | https://pubchem.ncbi.nlm.nih.gov | |
Description | Data deposited in or computed by PubChem | |
Molecular Weight |
229.19 g/mol |
Source
|
Source | PubChem | |
URL | https://pubchem.ncbi.nlm.nih.gov | |
Description | Data deposited in or computed by PubChem | |
Disclaimer and Information on In-Vitro Research Products
Please be aware that all articles and product information presented on BenchChem are intended solely for informational purposes. The products available for purchase on BenchChem are specifically designed for in-vitro studies, which are conducted outside of living organisms. In-vitro studies, derived from the Latin term "in glass," involve experiments performed in controlled laboratory settings using cells or tissues. It is important to note that these products are not categorized as medicines or drugs, and they have not received approval from the FDA for the prevention, treatment, or cure of any medical condition, ailment, or disease. We must emphasize that any form of bodily introduction of these products into humans or animals is strictly prohibited by law. It is essential to adhere to these guidelines to ensure compliance with legal and ethical standards in research and experimentation.