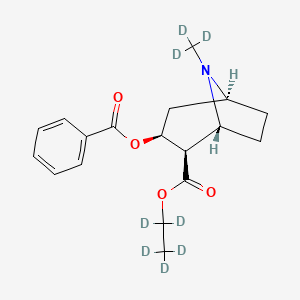
Cocaethylene-D8
Overview
Description
Cocaethylene-D8, also known as this compound, is a deuterated analog of benzoylecgonine ethyl ester. It is a metabolite of cocaine formed in the presence of ethanol. This compound is often used as a stable-labeled internal standard for quantitation of cocaethylene levels in biological samples such as urine, blood, and saliva by liquid chromatography-mass spectrometry (LC/MS) or gas chromatography-mass spectrometry (GC/MS) for urine drug testing, clinical toxicology, forensic analysis, or isotope dilution methods .
Preparation Methods
The preparation of benzoylecgonine ethyl ester-D8 involves the transesterification of cocaine with ethanol in the presence of deuterium. The synthetic route typically includes the following steps:
Hydrolysis of Cocaine: Cocaine is hydrolyzed to produce benzoylecgonine and methanol.
Transesterification: Benzoylecgonine undergoes transesterification with ethanol in the presence of deuterium to form benzoylecgonine ethyl ester-D8.
Chemical Reactions Analysis
Cocaethylene-D8 undergoes various chemical reactions, including:
Oxidation: This compound can be oxidized to form benzoylecgonine.
Reduction: Reduction reactions can convert benzoylecgonine ethyl ester-D8 back to its parent compound, cocaine.
Substitution: Substitution reactions can occur at the ester group, leading to the formation of different ester derivatives.
Common reagents and conditions used in these reactions include oxidizing agents like potassium permanganate, reducing agents like lithium aluminum hydride, and various alcohols for esterification reactions. The major products formed from these reactions include benzoylecgonine, cocaine, and other ester derivatives .
Scientific Research Applications
Cocaethylene-D8 has several scientific research applications:
Chemistry: It is used as a reference standard in analytical chemistry for the quantitation of cocaethylene levels in biological samples.
Biology: The compound is used in studies related to the metabolism of cocaine and ethanol in the human body.
Medicine: It is utilized in clinical toxicology to monitor cocaine and ethanol abuse.
Mechanism of Action
Cocaethylene-D8 exerts its effects by inhibiting the action of the serotonin transporter, norepinephrine transporter, and dopamine transporter. This inhibition increases the levels of serotonergic, noradrenergic, and dopaminergic neurotransmission in the brain.
Comparison with Similar Compounds
Cocaethylene-D8 is structurally similar to other cocaine metabolites, such as:
Benzoylecgonine: The major metabolite of cocaine formed by hydrolysis.
Ecgonine methyl ester: Another metabolite of cocaine formed by hydrolysis.
Cocaethylene: The ethyl ester of benzoylecgonine formed in the presence of ethanol
The uniqueness of benzoylecgonine ethyl ester-D8 lies in its deuterated form, which makes it a stable-labeled internal standard suitable for precise quantitation in analytical methods .
Biological Activity
Cocaethylene-D8, a deuterated analog of cocaethylene, is a compound formed in the liver when cocaine and ethanol are consumed simultaneously. This article explores its biological activity, including pharmacokinetics, metabolic pathways, and its effects on health, particularly focusing on its neurochemical properties and implications for substance abuse.
Cocaethylene is produced when cocaine and ethanol coexist in the bloodstream. This process involves the transesterification of cocaine with ethanol, leading to the formation of cocaethylene instead of the typical metabolites like benzoylecgonine. The presence of cocaethylene in biological systems has been associated with significant psychoactive effects and potential health risks.
2. Pharmacokinetics
The pharmacokinetic profile of this compound can be summarized as follows:
Parameter | Cocaine | Cocaethylene |
---|---|---|
Clearance (L/min) | 0.91 ± 0.22 | 0.79 ± 0.16 |
Volume Distribution (L/kg) | 2.6 ± 0.82 | 2.7 ± 0.47 |
These values indicate that cocaethylene has a slightly lower clearance rate compared to cocaine, suggesting a prolonged presence in the body when both substances are consumed together .
3. Metabolism
Cocaethylene is primarily metabolized by carboxylesterase enzymes in the liver, particularly hCE1 and hCE2. These enzymes facilitate the hydrolysis of cocaine into inactive metabolites under normal circumstances; however, in the presence of ethanol, they catalyze the formation of cocaethylene . This metabolic pathway highlights the biochemical interactions between cocaine and ethanol and their implications for toxicity.
4.1 Neurochemical Effects
Cocaethylene acts as a potent reuptake inhibitor for serotonin, norepinephrine, and dopamine transporters, classifying it as a serotonin-norepinephrine-dopamine reuptake inhibitor (SNDRI). This mechanism can lead to increased levels of these neurotransmitters in the brain, contributing to its euphoric effects .
4.2 Cardiovascular Impact
Research indicates that cocaethylene may have more severe cardiovascular effects compared to cocaine alone due to its enhanced sodium channel blocking properties. This can result in increased heart rates and blood pressure following consumption .
5.1 Liver Health
The presence of cocaethylene has been linked to increased risks of liver fibrosis among users of cocaine and alcohol. A study indicated that participants with detectable levels of cocaethylene had more than three times the odds of developing liver fibrosis compared to non-users .
5.2 Trauma Cases
In trauma settings, patients with cocaethylene present in their systems were significantly more likely to require intensive care unit (ICU) admission, indicating its association with severe health outcomes following substance use .
6. Case Studies
-
Case Study 1: Trauma Patients
A study involving 417 trauma patients found that 8.9% had cocaethylene metabolites present, correlating with higher ICU admission rates (odds ratio = 5.9) . -
Case Study 2: Liver Fibrosis
Out of 649 participants analyzed for substance use, those with cocaethylene had significantly higher concentrations of cocaine metabolites and were more likely to engage in hazardous drinking behaviors .
7. Conclusion
This compound exhibits significant biological activity through its interaction with neurotransmitter systems and its potential for adverse health effects, particularly concerning liver function and cardiovascular health. Understanding these effects is crucial for addressing issues related to polysubstance use involving cocaine and alcohol.
Properties
IUPAC Name |
1,1,2,2,2-pentadeuterioethyl (1R,2R,3S,5S)-3-benzoyloxy-8-(trideuteriomethyl)-8-azabicyclo[3.2.1]octane-2-carboxylate | |
---|---|---|
Source | PubChem | |
URL | https://pubchem.ncbi.nlm.nih.gov | |
Description | Data deposited in or computed by PubChem | |
InChI |
InChI=1S/C18H23NO4/c1-3-22-18(21)16-14-10-9-13(19(14)2)11-15(16)23-17(20)12-7-5-4-6-8-12/h4-8,13-16H,3,9-11H2,1-2H3/t13-,14+,15-,16+/m0/s1/i1D3,2D3,3D2 | |
Source | PubChem | |
URL | https://pubchem.ncbi.nlm.nih.gov | |
Description | Data deposited in or computed by PubChem | |
InChI Key |
NMPOSNRHZIWLLL-DKCRSESOSA-N | |
Source | PubChem | |
URL | https://pubchem.ncbi.nlm.nih.gov | |
Description | Data deposited in or computed by PubChem | |
Canonical SMILES |
CCOC(=O)C1C2CCC(N2C)CC1OC(=O)C3=CC=CC=C3 | |
Source | PubChem | |
URL | https://pubchem.ncbi.nlm.nih.gov | |
Description | Data deposited in or computed by PubChem | |
Isomeric SMILES |
[2H]C([2H])([2H])C([2H])([2H])OC(=O)[C@@H]1[C@H]2CC[C@H](N2C([2H])([2H])[2H])C[C@@H]1OC(=O)C3=CC=CC=C3 | |
Source | PubChem | |
URL | https://pubchem.ncbi.nlm.nih.gov | |
Description | Data deposited in or computed by PubChem | |
Molecular Formula |
C18H23NO4 | |
Source | PubChem | |
URL | https://pubchem.ncbi.nlm.nih.gov | |
Description | Data deposited in or computed by PubChem | |
DSSTOX Substance ID |
DTXSID601043108 | |
Record name | Cocaethylene-D8 | |
Source | EPA DSSTox | |
URL | https://comptox.epa.gov/dashboard/DTXSID601043108 | |
Description | DSSTox provides a high quality public chemistry resource for supporting improved predictive toxicology. | |
Molecular Weight |
325.4 g/mol | |
Source | PubChem | |
URL | https://pubchem.ncbi.nlm.nih.gov | |
Description | Data deposited in or computed by PubChem | |
CAS No. |
152521-09-0 | |
Record name | Cocaethylene-D8 | |
Source | EPA DSSTox | |
URL | https://comptox.epa.gov/dashboard/DTXSID601043108 | |
Description | DSSTox provides a high quality public chemistry resource for supporting improved predictive toxicology. | |
Record name | 152521-09-0 | |
Source | European Chemicals Agency (ECHA) | |
URL | https://echa.europa.eu/information-on-chemicals | |
Description | The European Chemicals Agency (ECHA) is an agency of the European Union which is the driving force among regulatory authorities in implementing the EU's groundbreaking chemicals legislation for the benefit of human health and the environment as well as for innovation and competitiveness. | |
Explanation | Use of the information, documents and data from the ECHA website is subject to the terms and conditions of this Legal Notice, and subject to other binding limitations provided for under applicable law, the information, documents and data made available on the ECHA website may be reproduced, distributed and/or used, totally or in part, for non-commercial purposes provided that ECHA is acknowledged as the source: "Source: European Chemicals Agency, http://echa.europa.eu/". Such acknowledgement must be included in each copy of the material. ECHA permits and encourages organisations and individuals to create links to the ECHA website under the following cumulative conditions: Links can only be made to webpages that provide a link to the Legal Notice page. | |
Disclaimer and Information on In-Vitro Research Products
Please be aware that all articles and product information presented on BenchChem are intended solely for informational purposes. The products available for purchase on BenchChem are specifically designed for in-vitro studies, which are conducted outside of living organisms. In-vitro studies, derived from the Latin term "in glass," involve experiments performed in controlled laboratory settings using cells or tissues. It is important to note that these products are not categorized as medicines or drugs, and they have not received approval from the FDA for the prevention, treatment, or cure of any medical condition, ailment, or disease. We must emphasize that any form of bodily introduction of these products into humans or animals is strictly prohibited by law. It is essential to adhere to these guidelines to ensure compliance with legal and ethical standards in research and experimentation.