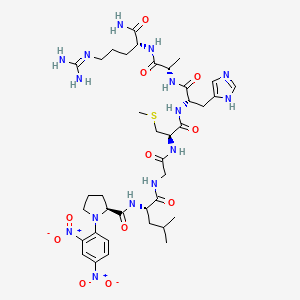
Dnp-pro-leu-gly-cys(ME)-his-ala-D-arg-NH2
- Click on QUICK INQUIRY to receive a quote from our team of experts.
- With the quality product at a COMPETITIVE price, you can focus more on your research.
Overview
Description
The compound Dnp-pro-leu-gly-cys(ME)-his-ala-D-arg-NH2 is a synthetic peptide substrate designed for specific enzymatic studies. It is particularly used as a substrate for matrix metalloproteinase-1 (MMP-1), which is an enzyme involved in the breakdown of extracellular matrix components. The compound’s structure includes a dinitrophenyl (Dnp) group, which serves as a chromophore, allowing for the monitoring of enzymatic activity through spectrophotometric methods .
Mechanism of Action
Target of Action
The primary target of Dnp-Pro-Leu-Gly-Cys(Me)-His-Ala-D-Arg-NH2 is Matrix Metalloproteinase-1 (MMP-1) . MMP-1 is an enzyme that plays a crucial role in the degradation of extracellular matrix components, which is essential for tissue remodeling and repair.
Mode of Action
This compound acts as a substrate for MMP-1 . It has been designed to optimize substrate specificity, with two amino acids substituted at the cleavage site of the collagenase substrate . This modification allows the compound to have a 10-fold better kcat/Km value for human fibroblast collagenase than the FRET substrate M-1855 .
Biochemical Pathways
The interaction of this compound with MMP-1 affects the collagen degradation pathway . By acting as a substrate for MMP-1, it influences the rate at which this enzyme can break down collagen, a key component of the extracellular matrix. This can have downstream effects on processes such as tissue remodeling and wound healing.
Pharmacokinetics
It is soluble in DMSO , which can facilitate its delivery into biological systems.
Biochemical Analysis
Biochemical Properties
Dnp-pro-leu-gly-cys(ME)-his-ala-D-arg-NH2 interacts with several enzymes, proteins, and other biomolecules. It has been found to have a 10-fold better kcat/Km value for human fibroblast collagenase than the FRET substrate M-1855 . This suggests that this compound has a high affinity for this enzyme and can be efficiently catalyzed.
Molecular Mechanism
It is known to interact with human fibroblast collagenase, suggesting it may play a role in the breakdown of collagen in the extracellular matrix
Preparation Methods
Synthetic Routes and Reaction Conditions
The synthesis of Dnp-pro-leu-gly-cys(ME)-his-ala-D-arg-NH2 involves solid-phase peptide synthesis (SPPS), a common method for producing peptides. The process typically includes the following steps:
Resin Loading: The synthesis begins with the attachment of the first amino acid to a solid resin.
Coupling: Subsequent amino acids are sequentially added using coupling reagents such as N,N’-diisopropylcarbodiimide (DIC) and hydroxybenzotriazole (HOBt).
Deprotection: After each coupling step, the protecting groups on the amino acids are removed using trifluoroacetic acid (TFA).
Cleavage: Once the peptide chain is complete, it is cleaved from the resin using a cleavage cocktail containing TFA, water, and scavengers like triisopropylsilane (TIS).
Purification: The crude peptide is purified using high-performance liquid chromatography (HPLC).
Industrial Production Methods
Industrial production of this peptide follows similar steps but on a larger scale. Automation and optimization of SPPS allow for the efficient production of high-purity peptides. The use of advanced purification techniques, such as preparative HPLC, ensures the consistency and quality of the final product .
Chemical Reactions Analysis
Types of Reactions
Dnp-pro-leu-gly-cys(ME)-his-ala-D-arg-NH2: undergoes several types of chemical reactions, including:
Oxidation: The cysteine residue can undergo oxidation to form disulfide bonds.
Reduction: Disulfide bonds can be reduced back to free thiol groups using reducing agents like dithiothreitol (DTT).
Substitution: The dinitrophenyl group can participate in nucleophilic substitution reactions.
Common Reagents and Conditions
Oxidation: Hydrogen peroxide (H2O2) or iodine (I2) can be used as oxidizing agents.
Reduction: Dithiothreitol (DTT) or tris(2-carboxyethyl)phosphine (TCEP) are common reducing agents.
Substitution: Nucleophiles such as amines or thiols can react with the dinitrophenyl group under mild conditions.
Major Products
Oxidation: Formation of disulfide-linked peptides.
Reduction: Regeneration of free thiol-containing peptides.
Substitution: Formation of substituted dinitrophenyl derivatives
Scientific Research Applications
Dnp-pro-leu-gly-cys(ME)-his-ala-D-arg-NH2: is widely used in scientific research, particularly in the following areas:
Chemistry: As a substrate for studying the activity of matrix metalloproteinases (MMPs), which are involved in various biochemical processes.
Biology: Used in enzymatic assays to monitor the activity of MMP-1 and other related enzymes.
Medicine: Research on the role of MMPs in diseases such as cancer, arthritis, and cardiovascular diseases.
Industry: Employed in the development of diagnostic assays and therapeutic agents targeting MMPs
Comparison with Similar Compounds
Dnp-pro-leu-gly-cys(ME)-his-ala-D-arg-NH2: is unique due to its specific sequence and the presence of the dinitrophenyl group. Similar compounds include:
Dnp-pro-leu-gly-leu-trp-ala-D-arg-NH2: Another substrate for MMP-1 with a different sequence.
Dnp-pro-leu-gly-cys-his-ala-D-arg-NH2: Lacks the methyl group on cysteine.
Dnp-pro-leu-gly-cys(ME)-his-ala-D-arg-OH: Has a free carboxyl group instead of an amide group at the C-terminus.
These compounds differ in their sequences and functional groups, which can affect their specificity and reactivity with MMP-1 .
Properties
IUPAC Name |
(2S)-N-[(2S)-1-[[2-[[(2R)-1-[[(2S)-1-[[(2S)-1-[[(2R)-1-amino-5-(diaminomethylideneamino)-1-oxopentan-2-yl]amino]-1-oxopropan-2-yl]amino]-3-(1H-imidazol-5-yl)-1-oxopropan-2-yl]amino]-3-methylsulfanyl-1-oxopropan-2-yl]amino]-2-oxoethyl]amino]-4-methyl-1-oxopentan-2-yl]-1-(2,4-dinitrophenyl)pyrrolidine-2-carboxamide |
Source
|
---|---|---|
Source | PubChem | |
URL | https://pubchem.ncbi.nlm.nih.gov | |
Description | Data deposited in or computed by PubChem | |
InChI |
InChI=1S/C38H57N15O11S/c1-20(2)13-25(50-37(60)29-8-6-12-51(29)28-10-9-23(52(61)62)15-30(28)53(63)64)34(57)44-17-31(54)47-27(18-65-4)36(59)49-26(14-22-16-42-19-45-22)35(58)46-21(3)33(56)48-24(32(39)55)7-5-11-43-38(40)41/h9-10,15-16,19-21,24-27,29H,5-8,11-14,17-18H2,1-4H3,(H2,39,55)(H,42,45)(H,44,57)(H,46,58)(H,47,54)(H,48,56)(H,49,59)(H,50,60)(H4,40,41,43)/t21-,24+,25-,26-,27-,29-/m0/s1 |
Source
|
Source | PubChem | |
URL | https://pubchem.ncbi.nlm.nih.gov | |
Description | Data deposited in or computed by PubChem | |
InChI Key |
RQIDWDWPFOGZMH-YPJPCPJPSA-N |
Source
|
Source | PubChem | |
URL | https://pubchem.ncbi.nlm.nih.gov | |
Description | Data deposited in or computed by PubChem | |
Canonical SMILES |
CC(C)CC(C(=O)NCC(=O)NC(CSC)C(=O)NC(CC1=CN=CN1)C(=O)NC(C)C(=O)NC(CCCN=C(N)N)C(=O)N)NC(=O)C2CCCN2C3=C(C=C(C=C3)[N+](=O)[O-])[N+](=O)[O-] |
Source
|
Source | PubChem | |
URL | https://pubchem.ncbi.nlm.nih.gov | |
Description | Data deposited in or computed by PubChem | |
Isomeric SMILES |
C[C@@H](C(=O)N[C@H](CCCN=C(N)N)C(=O)N)NC(=O)[C@H](CC1=CN=CN1)NC(=O)[C@H](CSC)NC(=O)CNC(=O)[C@H](CC(C)C)NC(=O)[C@@H]2CCCN2C3=C(C=C(C=C3)[N+](=O)[O-])[N+](=O)[O-] |
Source
|
Source | PubChem | |
URL | https://pubchem.ncbi.nlm.nih.gov | |
Description | Data deposited in or computed by PubChem | |
Molecular Formula |
C38H57N15O11S |
Source
|
Source | PubChem | |
URL | https://pubchem.ncbi.nlm.nih.gov | |
Description | Data deposited in or computed by PubChem | |
Molecular Weight |
932.0 g/mol |
Source
|
Source | PubChem | |
URL | https://pubchem.ncbi.nlm.nih.gov | |
Description | Data deposited in or computed by PubChem | |
Disclaimer and Information on In-Vitro Research Products
Please be aware that all articles and product information presented on BenchChem are intended solely for informational purposes. The products available for purchase on BenchChem are specifically designed for in-vitro studies, which are conducted outside of living organisms. In-vitro studies, derived from the Latin term "in glass," involve experiments performed in controlled laboratory settings using cells or tissues. It is important to note that these products are not categorized as medicines or drugs, and they have not received approval from the FDA for the prevention, treatment, or cure of any medical condition, ailment, or disease. We must emphasize that any form of bodily introduction of these products into humans or animals is strictly prohibited by law. It is essential to adhere to these guidelines to ensure compliance with legal and ethical standards in research and experimentation.