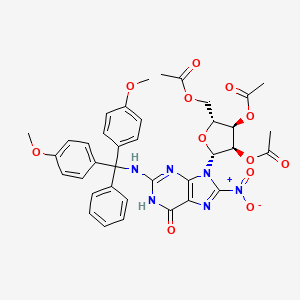
N-(4,4'-Dimethoxytrityl)-8-nitroguanosine 2',3',5'-Triacetate
Overview
Description
N-(4,4’-Dimethoxytrityl)-8-nitroguanosine 2’,3’,5’-Triacetate is a chemically modified nucleoside derivative. It is primarily used in the field of nucleic acid chemistry, particularly in the synthesis of oligonucleotides. The compound features a protective group, the 4,4’-dimethoxytrityl group, which is commonly used to protect the hydroxyl groups of nucleosides during chemical synthesis.
Preparation Methods
Synthetic Routes and Reaction Conditions: The synthesis of N-(4,4’-Dimethoxytrityl)-8-nitroguanosine 2’,3’,5’-Triacetate typically involves multiple steps:
Protection of Guanosine: The guanosine molecule is first protected by the 4,4’-dimethoxytrityl group. This is achieved by reacting guanosine with 4,4’-dimethoxytrityl chloride in the presence of a base such as pyridine.
Nitration: The protected guanosine is then nitrated at the 8-position using a nitrating agent like nitric acid or a mixture of sulfuric acid and nitric acid.
Acetylation: Finally, the hydroxyl groups at the 2’, 3’, and 5’ positions are acetylated using acetic anhydride in the presence of a catalyst like pyridine.
Industrial Production Methods: Industrial production of this compound follows similar synthetic routes but on a larger scale. The process involves careful control of reaction conditions to ensure high yield and purity. The use of automated synthesizers and purification systems like high-performance liquid chromatography (HPLC) is common in industrial settings.
Types of Reactions:
Oxidation: The nitro group in the compound can undergo reduction to form an amino group.
Substitution: The protective 4,4’-dimethoxytrityl group can be removed under acidic conditions, revealing the free hydroxyl groups.
Hydrolysis: The acetate groups can be hydrolyzed to yield the free hydroxyl groups.
Common Reagents and Conditions:
Reduction: Common reducing agents include hydrogen gas with a palladium catalyst or sodium dithionite.
Deprotection: Acidic conditions, such as treatment with trichloroacetic acid, are used to remove the 4,4’-dimethoxytrityl group.
Hydrolysis: Mild basic conditions, such as sodium bicarbonate, are used to hydrolyze the acetate groups.
Major Products:
Amino-derivative: Formed from the reduction of the nitro group.
Free nucleoside: Obtained after deprotection and hydrolysis steps.
Scientific Research Applications
Medicinal Chemistry
1.1 Anticancer Research
N-(4,4'-Dimethoxytrityl)-8-nitroguanosine 2',3',5'-triacetate has shown promise as a potential anticancer agent. Its structure allows for the selective targeting of cancer cells by exploiting the differences in cellular metabolism between normal and cancerous tissues. Studies have indicated that compounds with nitro groups can induce apoptosis in cancer cells through the generation of reactive oxygen species (ROS) .
Case Study:
A recent study demonstrated that this compound exhibited cytotoxic effects against various cancer cell lines, including breast and prostate cancer cells. The mechanism was attributed to the compound's ability to disrupt DNA synthesis and repair mechanisms in rapidly dividing cells .
Molecular Biology
2.1 Nucleic Acid Research
this compound serves as a valuable building block in oligonucleotide synthesis. Its protective groups facilitate the synthesis of modified RNA and DNA strands, which are essential for studying gene expression and regulation.
Data Table: Oligonucleotide Synthesis Applications
Nanotechnology
3.1 Nanocarriers for Drug Delivery
The compound can be utilized in the design of nanocarriers for targeted drug delivery systems. Its ability to form stable complexes with various therapeutic agents enhances the bioavailability and efficacy of drugs while minimizing side effects.
Case Study:
Research has shown that nanoparticles incorporating this compound can effectively deliver chemotherapeutic agents directly to tumor sites, improving treatment outcomes in preclinical models .
Mechanism of Action
The compound exerts its effects primarily through its incorporation into nucleic acid sequences. The protective groups allow for selective reactions at specific sites, facilitating the synthesis of complex oligonucleotides. The nitro group can be reduced to an amino group, which can participate in further chemical modifications or interactions with biological molecules.
Comparison with Similar Compounds
N-(4,4’-Dimethoxytrityl)-8-bromoguanosine 2’,3’,5’-Triacetate: Similar in structure but with a bromine atom instead of a nitro group.
N-(4,4’-Dimethoxytrityl)-8-aminoguanosine 2’,3’,5’-Triacetate: Contains an amino group instead of a nitro group.
Uniqueness: N-(4,4’-Dimethoxytrityl)-8-nitroguanosine 2’,3’,5’-Triacetate is unique due to the presence of the nitro group, which can be selectively reduced to an amino group, providing additional versatility in chemical synthesis and biological applications.
This compound’s unique properties and versatile applications make it a valuable tool in the fields of chemistry, biology, and medicine
Biological Activity
N-(4,4'-Dimethoxytrityl)-8-nitroguanosine 2',3',5'-triacetate is a modified nucleoside that has garnered attention due to its potential biological activities, particularly in the context of oxidative stress and DNA damage. This compound is a derivative of guanosine, where the nitro group at the 8-position of guanine introduces unique chemical properties that can influence its biological interactions.
Chemical Structure and Properties
The chemical structure of this compound can be represented as follows:
- Molecular Formula : C₁₈H₁₉N₅O₇
- Molecular Weight : 405.37 g/mol
This compound features a dimethoxytrityl group which enhances its stability and solubility in biological systems, allowing for better interaction with cellular components.
The biological activity of this compound primarily revolves around its ability to form adducts with DNA and RNA, leading to modifications that can trigger various cellular responses. The nitro group on the guanine moiety is known to participate in redox reactions, generating reactive oxygen species (ROS) that can further modify nucleic acids and proteins.
Key Mechanisms:
- Nitration of Guanine : The introduction of the nitro group at the 8-position leads to the formation of 8-nitroguanine, a known marker of oxidative DNA damage. This modification can interfere with base pairing during DNA replication, potentially leading to mutagenesis .
- Reactive Oxygen Species Generation : The compound can induce oxidative stress by generating ROS, which can damage cellular components including lipids, proteins, and nucleic acids. This is particularly relevant in inflammatory conditions where peroxynitrite is produced .
Biological Activities
Research has highlighted several biological activities associated with this compound:
- Genotoxicity : Studies have shown that this compound exhibits genotoxic effects in vitro, leading to increased mutation rates in bacterial models such as Escherichia coli when exposed to oxidative stress conditions .
- Cellular Signaling : The presence of 8-nitroguanosine derivatives has been implicated in modulating cyclic GMP (cGMP) signaling pathways. These pathways are crucial for various physiological processes including vasodilation and neurotransmission .
- Potential Therapeutic Applications : Due to its ability to modify nucleic acids and induce oxidative stress, there is interest in exploring this compound's role in cancer therapy, particularly in targeting tumor cells that exhibit high levels of oxidative stress .
Case Studies
Several studies have investigated the effects of nitrated guanosine derivatives:
- Study on Genotoxic Effects : A study demonstrated that exposure to peroxynitrite resulted in the formation of secondary lesions from 8-oxoGua derivatives, which were found to be highly mutagenic and genotoxic in vivo. This highlights the potential risks associated with compounds like N-(4,4'-Dimethoxytrityl)-8-nitroguanosine under oxidative conditions .
- Impact on Cellular Pathways : Another research effort focused on how nitrated guanine derivatives affect cGMP signaling pathways. The findings suggested that these modifications could lead to altered cellular responses during inflammatory processes .
Summary Table of Biological Activities
Q & A
Basic Research Questions
Q. What is the role of the 4,4'-dimethoxytrityl (DMT) group and triacetate protection in this compound during oligonucleotide synthesis?
The DMT group acts as a temporary protective group for the 5'-hydroxyl during solid-phase oligonucleotide synthesis. It enables selective deprotection under mild acidic conditions (e.g., 3% trichloroacetic acid in dichloromethane), facilitating stepwise chain elongation. The 2',3',5'-triacetate groups provide permanent protection for ribose hydroxyls, preventing unwanted side reactions during phosphoramidite coupling steps. This dual protection strategy ensures high coupling efficiency and minimizes premature chain termination .
Q. How is this compound purified after synthesis, and what analytical methods validate its purity?
Purification typically involves silica gel chromatography using gradients of hexane/ethyl acetate with 0.5% triethylamine to neutralize acidic byproducts. Final purity is assessed via reverse-phase HPLC (C18 column, acetonitrile/water gradient) and mass spectrometry (ESI-MS or MALDI-TOF). The DMT group’s UV absorbance at 498 nm allows for trityl yield monitoring during synthesis .
Q. What are the critical storage conditions to maintain the compound’s stability?
Store at 2–8°C under anhydrous conditions in amber vials to prevent hydrolysis of the acetyl groups and DMT cleavage. Desiccants (e.g., molecular sieves) are recommended for long-term storage. Stability tests under accelerated degradation conditions (40°C, 75% humidity) should be performed to establish shelf-life .
Advanced Research Questions
Q. How does the 8-nitro modification on guanosine impact duplex stability and enzymatic processing in antisense oligonucleotides?
The 8-nitro substituent introduces steric hindrance and electronic effects, potentially altering base-pairing fidelity. Studies using thermal denaturation (UV melting curves) and circular dichroism (CD) spectroscopy are required to assess duplex stability. Enzymatic resistance can be evaluated via exonuclease digestion assays (e.g., snake venom phosphodiesterase), with comparisons to unmodified guanosine controls .
Q. What synthetic challenges arise when scaling up the production of this phosphoramidite, and how are they addressed?
Key challenges include:
- Byproduct formation : Nitro group reduction during prolonged reactions. Use inert atmospheres (argon/nitrogen) and minimize reaction times.
- Purification scalability : Replace silica chromatography with flash chromatography or recrystallization for higher yields.
- Moisture sensitivity : Implement strict anhydrous protocols (e.g., Schlenk line techniques) .
Q. Can this compound be used to study site-specific DNA adducts, and what analytical techniques are suitable for detection?
Yes, the 8-nitro group can act as a reactive site for adduct formation with DNA-damaging agents (e.g., alkylators). Post-adduct synthesis, characterize products using:
- LC-MS/MS for mass confirmation.
- 32P-postlabeling for ultrasensitive detection of adducts in biological matrices.
- X-ray crystallography to resolve structural perturbations .
Q. Methodological Considerations
Q. How do competing protective group strategies (e.g., tert-butyldimethylsilyl vs. acetyl) affect the compound’s reactivity in RNA synthesis?
- Acetyl groups (used here) are stable under basic conditions but require harsh deprotection (e.g., ammonia/methylamine at 55°C).
- TBDMS groups offer orthogonal deprotection (fluoride ions) but may reduce coupling efficiency due to steric bulk.
Comparative studies using PAGE or HPLC analysis of crude oligonucleotides can optimize protection schemes .
Q. What protocols ensure efficient detritylation without damaging the 8-nitroguanosine moiety?
Use 3% dichloroacetic acid in dichloromethane (30-second treatments) instead of traditional trichloroacetic acid to minimize nitro group side reactions. Monitor detritylation efficiency via UV-vis at 498 nm and adjust acid concentration iteratively .
Properties
IUPAC Name |
[(2R,3R,4R,5R)-3,4-diacetyloxy-5-[2-[[bis(4-methoxyphenyl)-phenylmethyl]amino]-8-nitro-6-oxo-1H-purin-9-yl]oxolan-2-yl]methyl acetate | |
---|---|---|
Source | PubChem | |
URL | https://pubchem.ncbi.nlm.nih.gov | |
Description | Data deposited in or computed by PubChem | |
InChI |
InChI=1S/C37H36N6O12/c1-20(44)52-19-28-30(53-21(2)45)31(54-22(3)46)34(55-28)42-32-29(38-36(42)43(48)49)33(47)40-35(39-32)41-37(23-9-7-6-8-10-23,24-11-15-26(50-4)16-12-24)25-13-17-27(51-5)18-14-25/h6-18,28,30-31,34H,19H2,1-5H3,(H2,39,40,41,47)/t28-,30-,31-,34-/m1/s1 | |
Source | PubChem | |
URL | https://pubchem.ncbi.nlm.nih.gov | |
Description | Data deposited in or computed by PubChem | |
InChI Key |
NUZLZVNJVYBEJC-UTBAFCPYSA-N | |
Source | PubChem | |
URL | https://pubchem.ncbi.nlm.nih.gov | |
Description | Data deposited in or computed by PubChem | |
Canonical SMILES |
CC(=O)OCC1C(C(C(O1)N2C3=C(C(=O)NC(=N3)NC(C4=CC=CC=C4)(C5=CC=C(C=C5)OC)C6=CC=C(C=C6)OC)N=C2[N+](=O)[O-])OC(=O)C)OC(=O)C | |
Source | PubChem | |
URL | https://pubchem.ncbi.nlm.nih.gov | |
Description | Data deposited in or computed by PubChem | |
Isomeric SMILES |
CC(=O)OC[C@@H]1[C@H]([C@H]([C@@H](O1)N2C3=C(C(=O)NC(=N3)NC(C4=CC=CC=C4)(C5=CC=C(C=C5)OC)C6=CC=C(C=C6)OC)N=C2[N+](=O)[O-])OC(=O)C)OC(=O)C | |
Source | PubChem | |
URL | https://pubchem.ncbi.nlm.nih.gov | |
Description | Data deposited in or computed by PubChem | |
Molecular Formula |
C37H36N6O12 | |
Source | PubChem | |
URL | https://pubchem.ncbi.nlm.nih.gov | |
Description | Data deposited in or computed by PubChem | |
Molecular Weight |
756.7 g/mol | |
Source | PubChem | |
URL | https://pubchem.ncbi.nlm.nih.gov | |
Description | Data deposited in or computed by PubChem | |
Disclaimer and Information on In-Vitro Research Products
Please be aware that all articles and product information presented on BenchChem are intended solely for informational purposes. The products available for purchase on BenchChem are specifically designed for in-vitro studies, which are conducted outside of living organisms. In-vitro studies, derived from the Latin term "in glass," involve experiments performed in controlled laboratory settings using cells or tissues. It is important to note that these products are not categorized as medicines or drugs, and they have not received approval from the FDA for the prevention, treatment, or cure of any medical condition, ailment, or disease. We must emphasize that any form of bodily introduction of these products into humans or animals is strictly prohibited by law. It is essential to adhere to these guidelines to ensure compliance with legal and ethical standards in research and experimentation.