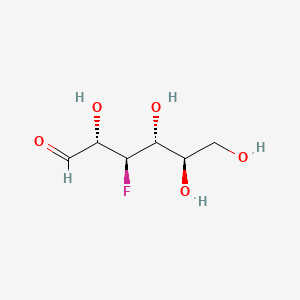
3-Deoxy-3-fluoro-D-allose
Overview
Description
3-Deoxy-3-fluoro-D-allose is a fluorinated sugar derivative, specifically a deoxyfluoro sugar It is a rare sugar that has been modified by replacing a hydroxyl group with a fluorine atom at the third carbon position
Mechanism of Action
Target of Action
The primary target of 3-Deoxy-3-fluoro-D-allose is the GLUT1 transporter . This transporter is responsible for the facilitated diffusion of glucose across the plasma membranes of mammalian cells .
Mode of Action
This compound interacts with its target, the GLUT1 transporter, in a similar manner to glucose . Its uptake by rat red blood cells (rbcs) was found to be significantly lower than that of glucose . The uptake of this compound by RBCs was inhibited by glucose and cytochalasin B, indicating the involvement of GLUT1-dependent transport .
Biochemical Pathways
Instead, it is preferentially utilized by aldose reductase and glucose dehydrogenase . This metabolism can be used as a marker for these particular enzyme activities .
Pharmacokinetics
The pharmacokinetic properties of this compound include high levels of kidney uptake and excretion, low serum protein binding, and high metabolic stability . These properties make it a preferable compound for renal imaging .
Result of Action
The result of the action of this compound is its high uptake in the kidneys, with minimal tracer accumulation in other organs . In vivo PET imaging visualized rapid excretion of the administered compound from the kidneys . It might be unsatisfactory for tumor imaging .
Action Environment
The action of this compound is influenced by environmental factors such as the presence of glucose and cytochalasin B, which can inhibit its uptake . Further validation studies in animal models are required to confirm the feasibility of this compound as a functional renal imaging agent .
Biochemical Analysis
Biochemical Properties
3-Deoxy-3-fluoro-D-allose interacts with various biomolecules in the body. For instance, it has been shown to be transported into rat red blood cells (RBCs) and its uptake was found to be inhibited by D-glucose and cytochalasin B, indicating the involvement of GLUT1-dependent transport . It was not phosphorylated by yeast hexokinase .
Cellular Effects
The effects of this compound on cells are quite significant. It has been shown to be taken up by cells in the brain, kidney, and liver . It does not inhibit the uptake of glucose by erythrocytes or the transport of glucose across cell membranes .
Molecular Mechanism
At the molecular level, this compound exerts its effects through various interactions. It is not metabolized through glycolysis or the pentose phosphate shunt . Instead, it is preferentially utilized by aldose reductase and glucose dehydrogenase . It is metabolized to 3-deoxy-3-fluoro-D-sorbitol (3-FS), via aldose reductase, and 3-deoxy-3-fluoro-D-fructose (3-FF), via the sorbitol dehydrogenase reaction with 3-FS, in rat cerebral tissue .
Temporal Effects in Laboratory Settings
In laboratory settings, the effects of this compound have been observed over time. The highest tissue uptake of this compound was observed in kidneys . Heart and brain uptake of this compound was below blood levels throughout the biodistribution studies (until 120 min after injection) .
Metabolic Pathways
This compound is involved in specific metabolic pathways. It is metabolized to 3-deoxy-3-fluoro-D-sorbitol (3-FS), via aldose reductase, and 3-deoxy-3-fluoro-D-fructose (3-FF), via the sorbitol dehydrogenase reaction with 3-FS, in rat cerebral tissue .
Transport and Distribution
This compound is transported and distributed within cells and tissues. It is taken up by cells in the brain, kidney, and liver . It does not inhibit the uptake of glucose by erythrocytes or the transport of glucose across cell membranes .
Preparation Methods
Synthetic Routes and Reaction Conditions
The synthesis of 3-Deoxy-3-fluoro-D-allose typically involves the fluorination of D-allose. One common method includes the use of 1,2,4,6-tetra-O-acetyl-3-O-trifluoromethanesulfonyl-β-D-glucopyranose as a precursor . The fluorination reaction is carried out under controlled conditions to ensure the selective introduction of the fluorine atom at the desired position.
Industrial Production Methods
While specific industrial production methods for this compound are not extensively documented, the general approach involves large-scale synthesis using similar fluorination techniques as those used in laboratory settings. The process may be optimized for higher yields and purity, with careful control of reaction parameters to ensure consistency and efficiency.
Chemical Reactions Analysis
Types of Reactions
3-Deoxy-3-fluoro-D-allose can undergo various chemical reactions, including:
Oxidation: The compound can be oxidized under specific conditions, leading to the formation of corresponding oxidized products.
Reduction: Reduction reactions can convert the compound into its reduced forms.
Substitution: The fluorine atom can be substituted with other functional groups under appropriate conditions.
Common Reagents and Conditions
Oxidation: Common oxidizing agents such as potassium permanganate or chromium trioxide can be used.
Reduction: Reducing agents like sodium borohydride or lithium aluminum hydride are typically employed.
Substitution: Nucleophilic substitution reactions may involve reagents like sodium azide or thiols.
Major Products Formed
The major products formed from these reactions depend on the specific conditions and reagents used. For example, oxidation may yield carboxylic acids or aldehydes, while reduction can produce alcohols or alkanes.
Scientific Research Applications
3-Deoxy-3-fluoro-D-allose has several scientific research applications, including:
Chemistry: Used as a building block in the synthesis of more complex molecules.
Biology: Studied for its interactions with biological systems, particularly in the context of metabolic pathways.
Medicine: Investigated for its potential as a diagnostic agent in positron emission tomography (PET) imaging.
Industry: Utilized in the development of novel materials and as a precursor in various chemical processes.
Comparison with Similar Compounds
Similar Compounds
3-Deoxy-3-fluoro-D-glucose: Another fluorinated sugar with similar structural modifications.
6-Deoxy-6-fluoro-D-allose: A compound with fluorination at the sixth carbon position.
2-Deoxy-2-fluoro-D-glucose: Widely used in PET imaging for its diagnostic properties.
Uniqueness
3-Deoxy-3-fluoro-D-allose is unique due to its specific fluorination at the third carbon position, which imparts distinct chemical and biological properties. This selective modification allows for targeted studies and applications, particularly in the fields of diagnostic imaging and metabolic research.
Properties
IUPAC Name |
(2S,3R,4R,5R)-3-fluoro-2,4,5,6-tetrahydroxyhexanal | |
---|---|---|
Source | PubChem | |
URL | https://pubchem.ncbi.nlm.nih.gov | |
Description | Data deposited in or computed by PubChem | |
InChI |
InChI=1S/C6H11FO5/c7-5(3(10)1-8)6(12)4(11)2-9/h1,3-6,9-12H,2H2/t3-,4+,5-,6+/m0/s1 | |
Source | PubChem | |
URL | https://pubchem.ncbi.nlm.nih.gov | |
Description | Data deposited in or computed by PubChem | |
InChI Key |
RMHCJIQOFXULDL-BGPJRJDNSA-N | |
Source | PubChem | |
URL | https://pubchem.ncbi.nlm.nih.gov | |
Description | Data deposited in or computed by PubChem | |
Canonical SMILES |
C(C(C(C(C(C=O)O)F)O)O)O | |
Source | PubChem | |
URL | https://pubchem.ncbi.nlm.nih.gov | |
Description | Data deposited in or computed by PubChem | |
Isomeric SMILES |
C([C@H]([C@H]([C@H]([C@H](C=O)O)F)O)O)O | |
Source | PubChem | |
URL | https://pubchem.ncbi.nlm.nih.gov | |
Description | Data deposited in or computed by PubChem | |
Molecular Formula |
C6H11FO5 | |
Source | PubChem | |
URL | https://pubchem.ncbi.nlm.nih.gov | |
Description | Data deposited in or computed by PubChem | |
DSSTOX Substance ID |
DTXSID70723577 | |
Record name | 3-Deoxy-3-fluoro-D-allose | |
Source | EPA DSSTox | |
URL | https://comptox.epa.gov/dashboard/DTXSID70723577 | |
Description | DSSTox provides a high quality public chemistry resource for supporting improved predictive toxicology. | |
Molecular Weight |
182.15 g/mol | |
Source | PubChem | |
URL | https://pubchem.ncbi.nlm.nih.gov | |
Description | Data deposited in or computed by PubChem | |
CAS No. |
99605-33-1 | |
Record name | 3-Deoxy-3-fluoro-D-allose | |
Source | EPA DSSTox | |
URL | https://comptox.epa.gov/dashboard/DTXSID70723577 | |
Description | DSSTox provides a high quality public chemistry resource for supporting improved predictive toxicology. | |
Disclaimer and Information on In-Vitro Research Products
Please be aware that all articles and product information presented on BenchChem are intended solely for informational purposes. The products available for purchase on BenchChem are specifically designed for in-vitro studies, which are conducted outside of living organisms. In-vitro studies, derived from the Latin term "in glass," involve experiments performed in controlled laboratory settings using cells or tissues. It is important to note that these products are not categorized as medicines or drugs, and they have not received approval from the FDA for the prevention, treatment, or cure of any medical condition, ailment, or disease. We must emphasize that any form of bodily introduction of these products into humans or animals is strictly prohibited by law. It is essential to adhere to these guidelines to ensure compliance with legal and ethical standards in research and experimentation.