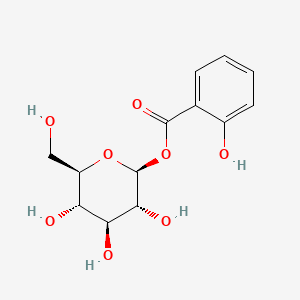
Glucosyl salicylate
Overview
Description
Glucosyl salicylate is a benzoate ester resulting from the formal condensation of the carboxy group of salicylic acid with the anomeric hydroxy group of D-glucose . This compound is known for its role as a plant metabolite and is classified as an O-acyl carbohydrate, D-glucoside, and benzoate ester . It is a white crystalline substance commonly found in nature and has garnered significant attention due to its diverse pharmaceutical properties and biological activities .
Preparation Methods
Synthetic Routes and Reaction Conditions: Glucosyl salicylate can be synthesized through chemical and enzymatic methods. One common chemical synthesis method involves the use of α-D-glucopyranosyl bromide as the glycosyl donor and salicylic acid as the acceptor . The reaction is typically carried out in the presence of a catalyst such as trifluoromethanesulfonic acid (TfOH) and an excess of dimethylformamide (DMF) to control the anomeric stereochemistry .
Industrial Production Methods: Industrial production of this compound often employs enzymatic synthesis due to its higher yield and environmentally friendly nature. Enzymes such as glycosyltransferases are used to catalyze the transfer of glucose from a donor molecule to salicylic acid . This method is preferred over chemical synthesis as it avoids the use of harsh chemicals and reduces the potential for environmental pollution .
Chemical Reactions Analysis
Types of Reactions: Glucosyl salicylate undergoes various chemical reactions, including:
Oxidation: It can be oxidized to form glucosyl salicylic acid derivatives.
Reduction: Reduction reactions can convert it into glucosyl salicyl alcohol.
Substitution: It can undergo nucleophilic substitution reactions where the glucose moiety is replaced by other functional groups.
Common Reagents and Conditions:
Oxidation: Common oxidizing agents include potassium permanganate (KMnO₄) and chromium trioxide (CrO₃).
Reduction: Reducing agents such as sodium borohydride (NaBH₄) and lithium aluminum hydride (LiAlH₄) are used.
Substitution: Nucleophiles like hydroxylamine (NH₂OH) and hydrazine (N₂H₄) are employed under mild acidic or basic conditions.
Major Products:
Oxidation: Glucosyl salicylic acid derivatives.
Reduction: Glucosyl salicyl alcohol.
Substitution: Various glucosyl derivatives depending on the nucleophile used.
Scientific Research Applications
Glucosyl salicylate has a wide range of applications in scientific research:
Mechanism of Action
Glucosyl salicylate exerts its effects primarily through the inhibition of cyclooxygenase (COX) enzymes, similar to other salicylates . By inhibiting COX-1 and COX-2, it reduces the conversion of arachidonic acid to prostaglandins and thromboxanes, thereby exerting anti-inflammatory and analgesic effects . Additionally, it may interact with other molecular targets and pathways involved in inflammation and pain signaling .
Comparison with Similar Compounds
Salicylic Acid: A precursor to glucosyl salicylate, known for its anti-inflammatory and keratolytic properties.
Methyl Salicylate: Another salicylate ester used topically for pain relief.
Acetylsalicylic Acid (Aspirin): A widely used analgesic and anti-inflammatory drug.
Uniqueness: this compound is unique due to its glycosylated structure, which enhances its solubility and stability compared to other salicylates . This glycosylation also imparts additional biological activities and improves its bioavailability .
Biological Activity
Glucosyl salicylate, a glucoside derivative of salicylic acid (SA), has garnered attention in recent research due to its significant biological activities, particularly in plant defense mechanisms and potential therapeutic applications. This article provides a comprehensive overview of the biological activity of this compound, supported by data tables, case studies, and detailed research findings.
Chemical Structure and Properties
This compound is characterized by the molecular formula and a molecular weight of 288.26 g/mol. It features a glucose moiety linked to the salicylic acid structure, which is crucial for its biological function. The compound is primarily studied for its role in plant defense responses and potential pharmacological effects in humans.
Plant Defense Mechanisms
This compound plays a critical role in the regulation of plant defense responses. It is involved in the glucosylation process mediated by specific glycosyltransferases, which modify salicylic acid to enhance its stability and reduce toxicity. The primary enzymes responsible for this modification include UGT74F1 and UGT74F2, which catalyze the formation of different glucosides from SA.
- UGT74F1 : Primarily forms salicylic acid glucoside (SAG).
- UGT74F2 : Produces both salicylic acid glucose ester (SGE) and SAG, with a higher specific activity for SGE formation.
This enzymatic activity modulates the levels of free SA within plants, influencing their susceptibility to pathogens such as Pseudomonas syringae .
Case Studies
- Glucosylation in Tobacco Plants : A study on Nicotiana tabacum demonstrated that glucosylation of SA leads to increased resistance against tobacco mosaic virus (TMV). The application of exogenous SA resulted in higher levels of this compound, suggesting a direct correlation between glucosylation and enhanced disease resistance .
- Metabolism Studies : Research involving pig skin models showed that topical application of formulations containing this compound resulted in significant dermal absorption and metabolism. After 24 hours, metabolites such as SA and benzoic acid were identified, indicating that this compound undergoes extensive first-pass metabolism .
Table 1: Enzymatic Activity of Glycosyltransferases
Enzyme | Product Formed | Specific Activity (µmol/min/mg) | Role in Plant Defense |
---|---|---|---|
UGT74F1 | Salicylic Acid Glucoside (SAG) | 0.5 | Increases resistance to pathogens |
UGT74F2 | Salicylic Acid Glucose Ester (SGE) | 5.0 | Modulates free SA levels |
Table 2: Metabolism of this compound in Pig Skin
Time Post-Application | Parent Compound Recovery (%) | Major Metabolites Detected |
---|---|---|
0 hours | 100 | None |
24 hours | 9.1 | Salicylic Acid, Benzoic Acid |
Q & A
Basic Research Questions
Q. What are the primary synthetic pathways for glucosyl salicylate, and how do reaction conditions influence yield and purity?
this compound is typically synthesized via enzymatic glycosylation or chemical esterification. Enzymatic methods (e.g., using glycosyltransferases) offer high specificity but require optimized pH, temperature, and cofactor availability . Chemical synthesis, such as transesterification between salicylic acid and glucose derivatives, often employs catalysts like sulfuric acid or lipases. Yield and purity depend on solvent choice (polar aprotic solvents enhance reactivity), reaction time, and purification steps (e.g., column chromatography or recrystallization). Impurities from incomplete esterification or side reactions (e.g., hydrolysis) necessitate rigorous analytical validation .
Q. Which analytical techniques are most reliable for quantifying this compound in biological samples?
High-performance liquid chromatography (HPLC) coupled with UV detection (λ = 300 nm) is widely used due to its sensitivity for aromatic structures. For complex matrices (e.g., plant extracts or serum), liquid chromatography-mass spectrometry (LC-MS) provides superior specificity, distinguishing this compound from isomers like methyl salicylate. Validation parameters (linearity, LOD, LOQ) must adhere to ICH guidelines, with internal standards (e.g., deuterated salicylates) correcting for matrix effects .
Advanced Research Questions
Q. How do genetic variations in glycosyltransferase enzymes regulate this compound biosynthesis in plants?
In tomato studies, Non-Smoky Glucosyl Transferase 1 (NSGT1) polymorphisms significantly alter this compound levels by modulating substrate affinity. For example, haplotype IV of NSGT1 reduces enzymatic activity by 40%, leading to accumulation of non-glycosylated salicylates. Interaction with loci like Methylesterase (MES) further influences flux through competing pathways (e.g., methylation vs. glycosylation). CRISPR-Cas9 knockout models and GWAS panels can identify functional haplotypes and epistatic interactions .
Q. What mechanisms underlie the contradictory reports on this compound’s anti-inflammatory efficacy in preclinical models?
Discrepancies often arise from differences in experimental design:
- Dose-dependent effects : Low doses (≤10 μM) may potentiate COX-2 inhibition, while higher doses (>50 μM) exhibit off-target cytotoxicity .
- Model specificity : Murine macrophages show higher sensitivity than human cell lines due to species-specific metabolic pathways (e.g., β-glucuronidase activity).
- Bioavailability : Cutaneous absorption varies with ester side-chain length; this compound’s hydrophilic glucose moiety reduces skin permeation compared to lipophilic esters (e.g., ethylhexyl salicylate) . Standardizing in vitro assays (e.g., LPS-induced IL-6 suppression) and using pharmacokinetic profiling (AUC, Cmax) can resolve inconsistencies .
Q. Methodological Guidance
Q. How should researchers optimize experimental designs to study this compound’s role in plant defense signaling?
- Controlled elicitation : Apply jasmonic acid or pathogen mimics (e.g., chitosan) to induce salicylate production, then quantify this compound via LC-MS at timed intervals .
- Knockdown/overexpression models : Use RNAi or transgenic plants to silence/overexpress NSGT1 and measure systemic acquired resistance (SAR) via PR gene expression .
- Isotopic tracing : Incubate plants with ¹³C-labeled salicylic acid to track glycosylation kinetics and metabolite partitioning .
Q. What statistical approaches are recommended for analyzing contradictory data on this compound’s stability under varying pH conditions?
- Multivariate analysis : Principal component analysis (PCA) can identify pH-dependent degradation pathways (e.g., hydrolysis vs. oxidation) from LC-MS datasets .
- Accelerated stability testing : Use Arrhenius modeling to predict shelf-life at 25°C based on degradation rates observed at elevated temperatures (40–60°C) .
- Robustness testing : Apply Plackett-Burman designs to assess the impact of pH, temperature, and light exposure on stability .
Q. Data Interpretation & Validation
Q. How can researchers validate the specificity of this compound antibodies in immunohistochemical assays?
- Pre-absorption controls : Pre-incubate antibodies with excess this compound to confirm signal reduction.
- Cross-reactivity testing : Test against structurally similar compounds (e.g., methyl salicylate, salicin) using ELISA or Western blot.
- Knockout validation : Compare staining in wild-type vs. NSGT1-knockout plant tissues .
Q. What criteria should guide the selection of in vivo models for studying this compound pharmacokinetics?
- Metabolic competency : Rodent models with humanized cytochrome P450 enzymes better predict human metabolism.
- Tissue-specific expression : Use transgenic reporters (e.g., GFP-tagged glucosyltransferases) to map biodistribution.
- Dose calibration : Align administered doses with physiologically relevant concentrations (e.g., mimicking dietary or topical exposure) .
Properties
IUPAC Name |
[(2S,3R,4S,5S,6R)-3,4,5-trihydroxy-6-(hydroxymethyl)oxan-2-yl] 2-hydroxybenzoate | |
---|---|---|
Source | PubChem | |
URL | https://pubchem.ncbi.nlm.nih.gov | |
Description | Data deposited in or computed by PubChem | |
InChI |
InChI=1S/C13H16O8/c14-5-8-9(16)10(17)11(18)13(20-8)21-12(19)6-3-1-2-4-7(6)15/h1-4,8-11,13-18H,5H2/t8-,9-,10+,11-,13+/m1/s1 | |
Source | PubChem | |
URL | https://pubchem.ncbi.nlm.nih.gov | |
Description | Data deposited in or computed by PubChem | |
InChI Key |
XNHKMZHWRNMFCU-HMUNZLOLSA-N | |
Source | PubChem | |
URL | https://pubchem.ncbi.nlm.nih.gov | |
Description | Data deposited in or computed by PubChem | |
Canonical SMILES |
C1=CC=C(C(=C1)C(=O)OC2C(C(C(C(O2)CO)O)O)O)O | |
Source | PubChem | |
URL | https://pubchem.ncbi.nlm.nih.gov | |
Description | Data deposited in or computed by PubChem | |
Isomeric SMILES |
C1=CC=C(C(=C1)C(=O)O[C@H]2[C@@H]([C@H]([C@@H]([C@H](O2)CO)O)O)O)O | |
Source | PubChem | |
URL | https://pubchem.ncbi.nlm.nih.gov | |
Description | Data deposited in or computed by PubChem | |
Molecular Formula |
C13H16O8 | |
Source | PubChem | |
URL | https://pubchem.ncbi.nlm.nih.gov | |
Description | Data deposited in or computed by PubChem | |
DSSTOX Substance ID |
DTXSID70678795 | |
Record name | 1-O-(2-Hydroxybenzoyl)-beta-D-glucopyranose | |
Source | EPA DSSTox | |
URL | https://comptox.epa.gov/dashboard/DTXSID70678795 | |
Description | DSSTox provides a high quality public chemistry resource for supporting improved predictive toxicology. | |
Molecular Weight |
300.26 g/mol | |
Source | PubChem | |
URL | https://pubchem.ncbi.nlm.nih.gov | |
Description | Data deposited in or computed by PubChem | |
CAS No. |
60517-74-0 | |
Record name | 1-O-(2-Hydroxybenzoyl)-beta-D-glucopyranose | |
Source | EPA DSSTox | |
URL | https://comptox.epa.gov/dashboard/DTXSID70678795 | |
Description | DSSTox provides a high quality public chemistry resource for supporting improved predictive toxicology. | |
Disclaimer and Information on In-Vitro Research Products
Please be aware that all articles and product information presented on BenchChem are intended solely for informational purposes. The products available for purchase on BenchChem are specifically designed for in-vitro studies, which are conducted outside of living organisms. In-vitro studies, derived from the Latin term "in glass," involve experiments performed in controlled laboratory settings using cells or tissues. It is important to note that these products are not categorized as medicines or drugs, and they have not received approval from the FDA for the prevention, treatment, or cure of any medical condition, ailment, or disease. We must emphasize that any form of bodily introduction of these products into humans or animals is strictly prohibited by law. It is essential to adhere to these guidelines to ensure compliance with legal and ethical standards in research and experimentation.