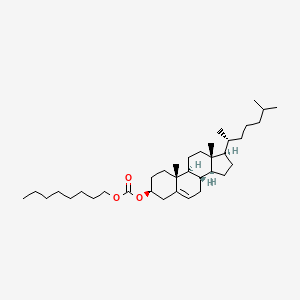
Cholesterol n-Octyl Carbonate
Overview
Description
Cholesterol n-Octyl Carbonate is a chemical compound with the molecular formula C36H62O3. It is an ester formed from cholesterol and n-octyl carbonate. This compound is known for its applications in various fields, including chemistry, biology, and medicine. It appears as a white to almost white powder or crystal at room temperature .
Scientific Research Applications
Cholesterol n-Octyl Carbonate has several applications in scientific research:
Chemistry: Used as a reagent in organic synthesis and as a building block for more complex molecules.
Biology: Employed in the study of lipid membranes and their interactions with proteins and other biomolecules.
Medicine: Investigated for its potential use in drug delivery systems, particularly in the formulation of liposomes and other lipid-based carriers.
Industry: Utilized in the production of cosmetics and personal care products due to its emollient properties
Future Directions
While specific future directions for Cholesterol n-Octyl Carbonate were not found in the search results, research into cholesterol-based compounds is ongoing. For instance, hybrid liposomes composed of cholesterol and other compounds are being investigated for potential use in drug delivery systems .
Mechanism of Action
Target of Action
Cholesterol n-Octyl Carbonate is a biochemical compound used in proteomics research . It is known to interact with proteins such as NPC1 and NPC2 , which are involved in the intralysosomal transport and efflux of cholesterol . These proteins play a crucial role in maintaining cellular cholesterol levels and ensuring cell viability, growth, and proliferation .
Mode of Action
The compound’s interaction with its targets involves the binding of cholesterol to the NTD of the NPC1 protein . This binding process is believed to be cooperative among NPC1 and NPC2 in cholesterol transport . The orientation of cholesterol binding to this NPC1 domain is opposite to the way that cholesterol binds to NPC2, supporting the notion that there is cooperation among NPC1 and NPC2 in cholesterol transport .
Biochemical Pathways
Cholesterol plays a critical role in multiple cellular functions, including regulating the physical properties of the plasma membrane, ensuring cell viability, growth, proliferation, and serving as a signaling and precursor molecule in biochemical pathways . Regulation of cellular cholesterol levels is tightly controlled via multiple pathways that include de-novo biosynthesis, uptake, recycling, and release . In particular, delivery of extracellular cholesterol to cells is achieved by receptor-mediated uptake of low-density lipoproteins (LDLs) that carry both free and esterified cholesterol .
Pharmacokinetics
It has been documented that lipid-based systems like hybrid membranes composed of 1,2-dipalmitoyl-sn-glycero-3-phosphocholine (dppc), cholesterol, and octyl-β-d-glucopyranoside (ogp) could have potential properties to use as nanovesicles for drug delivery .
Result of Action
The result of the compound’s action involves changes in the fluidity and compactness of the membrane occurring at temperatures of biological relevance . This is due to the synergistic action of cholesterol and OGP in polar–nonpolar spaces of the DPPC bilayer .
Action Environment
The action of this compound is influenced by environmental factors. For instance, the presence of OGP combined with cholesterol modifies thermodynamic parameters of membranes such as phase transition temperature, enthalpy change, and cooperativity . Furthermore, lipid vesicles containing OGP at 6.0 mM loaded with ibuprofen demonstrated good stability after 3 months of storage .
Preparation Methods
Synthetic Routes and Reaction Conditions: Cholesterol n-Octyl Carbonate can be synthesized through the esterification of cholesterol with n-octyl carbonate. The reaction typically involves the use of a catalyst such as dimethylaminopyridine (DMAP) and a dehydrating agent like dicyclohexylcarbodiimide (DCC). The reaction is carried out in an organic solvent such as chloroform or dichloromethane under reflux conditions .
Industrial Production Methods: In industrial settings, the production of this compound follows similar synthetic routes but on a larger scale. The process involves the careful control of reaction conditions to ensure high yield and purity. The product is then purified through recrystallization or chromatography techniques .
Chemical Reactions Analysis
Types of Reactions: Cholesterol n-Octyl Carbonate undergoes various chemical reactions, including:
Hydrolysis: In the presence of water and an acid or base catalyst, the ester bond can be hydrolyzed to yield cholesterol and n-octyl carbonate.
Oxidation: The compound can undergo oxidation reactions, particularly at the cholesterol moiety, leading to the formation of oxidized derivatives.
Substitution: The ester group can participate in nucleophilic substitution reactions, where the n-octyl group can be replaced by other nucleophiles.
Common Reagents and Conditions:
Hydrolysis: Acidic or basic conditions with water.
Oxidation: Oxidizing agents such as potassium permanganate or chromium trioxide.
Substitution: Nucleophiles like amines or alcohols under mild conditions.
Major Products Formed:
Hydrolysis: Cholesterol and n-octyl carbonate.
Oxidation: Oxidized cholesterol derivatives.
Substitution: Various substituted cholesterol derivatives.
Comparison with Similar Compounds
Cholesterol Oleyl Carbonate: Another ester of cholesterol, used in similar applications but with different physical properties.
Cholesterol Lauryl Carbonate: Similar in structure but with a lauryl group instead of an n-octyl group, leading to different solubility and interaction characteristics
Uniqueness: Cholesterol n-Octyl Carbonate is unique due to its specific interaction with lipid membranes, providing a balance between hydrophilic and hydrophobic properties. This makes it particularly useful in the formulation of stable liposomes and other lipid-based drug delivery systems .
Properties
IUPAC Name |
[(3S,8S,9S,10R,13R,14S,17R)-10,13-dimethyl-17-[(2R)-6-methylheptan-2-yl]-2,3,4,7,8,9,11,12,14,15,16,17-dodecahydro-1H-cyclopenta[a]phenanthren-3-yl] octyl carbonate | |
---|---|---|
Source | PubChem | |
URL | https://pubchem.ncbi.nlm.nih.gov | |
Description | Data deposited in or computed by PubChem | |
InChI |
InChI=1S/C36H62O3/c1-7-8-9-10-11-12-24-38-34(37)39-29-20-22-35(5)28(25-29)16-17-30-32-19-18-31(27(4)15-13-14-26(2)3)36(32,6)23-21-33(30)35/h16,26-27,29-33H,7-15,17-25H2,1-6H3/t27-,29+,30+,31-,32+,33+,35+,36-/m1/s1 | |
Source | PubChem | |
URL | https://pubchem.ncbi.nlm.nih.gov | |
Description | Data deposited in or computed by PubChem | |
InChI Key |
AUDCURYXLLEBIY-MKQVXYPISA-N | |
Source | PubChem | |
URL | https://pubchem.ncbi.nlm.nih.gov | |
Description | Data deposited in or computed by PubChem | |
Canonical SMILES |
CCCCCCCCOC(=O)OC1CCC2(C3CCC4(C(C3CC=C2C1)CCC4C(C)CCCC(C)C)C)C | |
Source | PubChem | |
URL | https://pubchem.ncbi.nlm.nih.gov | |
Description | Data deposited in or computed by PubChem | |
Isomeric SMILES |
CCCCCCCCOC(=O)O[C@H]1CC[C@@]2([C@H]3CC[C@]4([C@H]([C@@H]3CC=C2C1)CC[C@@H]4[C@H](C)CCCC(C)C)C)C | |
Source | PubChem | |
URL | https://pubchem.ncbi.nlm.nih.gov | |
Description | Data deposited in or computed by PubChem | |
Molecular Formula |
C36H62O3 | |
Source | PubChem | |
URL | https://pubchem.ncbi.nlm.nih.gov | |
Description | Data deposited in or computed by PubChem | |
DSSTOX Substance ID |
DTXSID00659875 | |
Record name | (3beta)-Cholest-5-en-3-yl octyl carbonate | |
Source | EPA DSSTox | |
URL | https://comptox.epa.gov/dashboard/DTXSID00659875 | |
Description | DSSTox provides a high quality public chemistry resource for supporting improved predictive toxicology. | |
Molecular Weight |
542.9 g/mol | |
Source | PubChem | |
URL | https://pubchem.ncbi.nlm.nih.gov | |
Description | Data deposited in or computed by PubChem | |
CAS No. |
15455-82-0 | |
Record name | Cholest-5-en-3-ol (3β)-, 3-(octyl carbonate) | |
Source | CAS Common Chemistry | |
URL | https://commonchemistry.cas.org/detail?cas_rn=15455-82-0 | |
Description | CAS Common Chemistry is an open community resource for accessing chemical information. Nearly 500,000 chemical substances from CAS REGISTRY cover areas of community interest, including common and frequently regulated chemicals, and those relevant to high school and undergraduate chemistry classes. This chemical information, curated by our expert scientists, is provided in alignment with our mission as a division of the American Chemical Society. | |
Explanation | The data from CAS Common Chemistry is provided under a CC-BY-NC 4.0 license, unless otherwise stated. | |
Record name | (3beta)-Cholest-5-en-3-yl octyl carbonate | |
Source | EPA DSSTox | |
URL | https://comptox.epa.gov/dashboard/DTXSID00659875 | |
Description | DSSTox provides a high quality public chemistry resource for supporting improved predictive toxicology. | |
Disclaimer and Information on In-Vitro Research Products
Please be aware that all articles and product information presented on BenchChem are intended solely for informational purposes. The products available for purchase on BenchChem are specifically designed for in-vitro studies, which are conducted outside of living organisms. In-vitro studies, derived from the Latin term "in glass," involve experiments performed in controlled laboratory settings using cells or tissues. It is important to note that these products are not categorized as medicines or drugs, and they have not received approval from the FDA for the prevention, treatment, or cure of any medical condition, ailment, or disease. We must emphasize that any form of bodily introduction of these products into humans or animals is strictly prohibited by law. It is essential to adhere to these guidelines to ensure compliance with legal and ethical standards in research and experimentation.