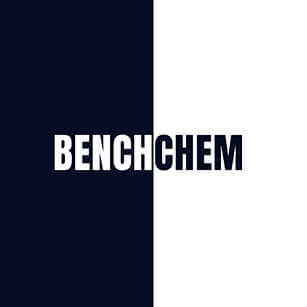
Tedizolid Phosphate Dimer
- Click on QUICK INQUIRY to receive a quote from our team of experts.
- With the quality product at a COMPETITIVE price, you can focus more on your research.
Overview
Description
Tedizolid phosphate dimer is a next-generation oxazolidinone antibiotic. It is a prodrug that is converted in vivo to its active form, tedizolid, which is effective against a variety of Gram-positive bacterial infections, including those caused by methicillin-resistant Staphylococcus aureus and vancomycin-resistant Enterococcus species . This compound has shown significant potency advantages over linezolid, another oxazolidinone antibiotic, particularly against microorganisms with reduced susceptibility to linezolid .
Mechanism of Action
Target of Action
Tedizolid phosphate dimer primarily targets the 50S subunit of the bacterial ribosome . This subunit plays a crucial role in protein synthesis, which is vital for bacterial growth and survival .
Mode of Action
Tedizolid inhibits bacterial protein synthesis by binding to the 50S subunit of the bacterial ribosome . This interaction disrupts the formation of a functional 70S initiation complex, which is an essential component of the translation process in bacteria . As a result, the bacterial cells are unable to synthesize proteins, leading to inhibition of bacterial growth .
Biochemical Pathways
The primary biochemical pathway affected by tedizolid is the protein synthesis pathway in bacteria . By binding to the 50S ribosomal subunit, tedizolid interferes with the translation process, preventing the assembly of amino acids into protein chains . This disruption in protein synthesis affects various downstream cellular processes, ultimately leading to bacterial cell death .
Pharmacokinetics
Tedizolid displays linear pharmacokinetics with good tissue penetration . It is administered as a phosphate prodrug that is converted to tedizolid, the active moiety . Prior to excretion, the majority of tedizolid is converted to an inactive sulphate conjugate in the liver . This conversion is unlikely to involve the action of cytochrome P450-family enzymes . Tedizolid has an apparent oral clearance of 6.9 ± 1.7 L/hr for a single dose and 8.4 ± 2.1 L/hr at steady-state .
Result of Action
The primary result of tedizolid’s action is the inhibition of bacterial growth . By disrupting protein synthesis, tedizolid prevents bacteria from producing essential proteins, leading to inhibition of bacterial growth and eventual bacterial cell death . Tedizolid is proven to be effective in the treatment of certain Gram-positive bacterial infections .
Action Environment
The efficacy and stability of tedizolid can be influenced by various environmental factors. For instance, the presence of granulocytes can augment its antibacterial effect .
Biochemical Analysis
Biochemical Properties
Tedizolid Phosphate Dimer plays a crucial role in biochemical reactions by inhibiting bacterial protein synthesis. It interacts with the 23S ribosomal RNA of the 50S subunit, preventing the formation of the initiation complex for protein synthesis . This interaction disrupts the translation process, leading to the inhibition of bacterial growth. This compound has shown high potency against methicillin-resistant Staphylococcus aureus and vancomycin-resistant Enterococcus faecium .
Cellular Effects
This compound affects various types of cells and cellular processes. It has been observed to influence cell function by inhibiting protein synthesis, which is essential for bacterial growth and replication . This inhibition leads to a decrease in bacterial cell viability and proliferation. Additionally, this compound has been shown to impact cell signaling pathways and gene expression by disrupting the translation process . This disruption can lead to changes in cellular metabolism and overall cell function.
Molecular Mechanism
The molecular mechanism of this compound involves its binding to the 23S ribosomal RNA of the 50S subunit of the bacterial ribosome . This binding prevents the formation of the initiation complex for protein synthesis, thereby inhibiting the translation process. This compound exerts its effects by blocking the peptidyl transferase center, which is essential for peptide bond formation during protein synthesis . This inhibition leads to the suppression of bacterial growth and replication.
Temporal Effects in Laboratory Settings
In laboratory settings, the effects of this compound have been observed to change over time. The compound has shown stability and sustained activity over extended periods . Studies have demonstrated that this compound maintains its antibacterial activity for up to 24 hours in in vitro models . Additionally, long-term studies have indicated that this compound does not degrade rapidly and retains its efficacy over time .
Dosage Effects in Animal Models
The effects of this compound vary with different dosages in animal models. Studies have shown that higher doses of this compound result in increased antibacterial activity . At very high doses, toxic or adverse effects have been observed, including hematological side effects such as thrombocytopenia . It is important to determine the optimal dosage to balance efficacy and safety in animal models.
Metabolic Pathways
This compound is involved in metabolic pathways that include its conversion to the active form, tedizolid, by phosphatases in vivo . The active form is then metabolized primarily in the liver, where it undergoes conjugation to form an inactive sulphate conjugate . This metabolic pathway ensures the elimination of the compound from the body while maintaining its antibacterial activity during treatment.
Transport and Distribution
This compound is transported and distributed within cells and tissues through passive diffusion and active transport mechanisms . It has been shown to penetrate tissues effectively, including skin and pulmonary tissues . The compound’s high tissue penetration is attributed to its ability to bind to plasma proteins and its favorable pharmacokinetic profile . This distribution ensures that this compound reaches the site of infection and exerts its antibacterial effects.
Subcellular Localization
The subcellular localization of this compound is primarily within the bacterial ribosome, where it binds to the 23S ribosomal RNA of the 50S subunit . This localization is crucial for its activity, as it allows the compound to inhibit protein synthesis effectively. Additionally, this compound may undergo post-translational modifications that enhance its targeting to specific cellular compartments . These modifications ensure that the compound reaches its site of action and exerts its antibacterial effects.
Preparation Methods
Synthetic Routes and Reaction Conditions: Tedizolid phosphate dimer is synthesized through a series of chemical reactions involving the formation of the oxazolidinone ring and the attachment of the phosphate group. The synthesis typically involves the following steps:
- Formation of the oxazolidinone core.
- Introduction of the phosphate group to form the prodrug .
Industrial Production Methods: Industrial production of this compound involves large-scale chemical synthesis under controlled conditions. The process includes:
- Use of high-purity reagents.
- Optimization of reaction conditions to maximize yield and purity.
- Implementation of purification techniques such as crystallization and chromatography to isolate the final product .
Chemical Reactions Analysis
Types of Reactions: Tedizolid phosphate dimer undergoes several types of chemical reactions, including:
Hydrolysis: The phosphate group is hydrolyzed in vivo to release the active form, tedizolid.
Oxidation and Reduction: These reactions are less common but can occur under specific conditions.
Common Reagents and Conditions:
Hydrolysis: Typically occurs in the presence of water and enzymes in the body.
Oxidation and Reduction: May involve reagents such as hydrogen peroxide or reducing agents like sodium borohydride under controlled laboratory conditions.
Major Products:
Scientific Research Applications
Tedizolid phosphate dimer has a wide range of scientific research applications, including:
Chemistry: Used as a model compound for studying the synthesis and reactivity of oxazolidinones.
Medicine: Used in the treatment of acute bacterial skin and skin structure infections.
Industry: Employed in the development of new antibiotics and in the study of drug resistance mechanisms.
Comparison with Similar Compounds
Daptomycin: A lipopeptide antibiotic with a different mechanism of action but used for similar types of infections.
Uniqueness of Tedizolid Phosphate Dimer:
Higher Potency: this compound is more potent than linezolid against Gram-positive bacteria, including resistant strains.
Favorable Pharmacokinetics: It has a longer half-life and better tissue penetration, allowing for once-daily dosing.
Reduced Adverse Effects: Lower total drug exposure reduces the risk of adverse effects such as thrombocytopenia.
This compound represents a significant advancement in the treatment of resistant bacterial infections, offering improved efficacy and safety profiles compared to other antibiotics in its class.
Properties
CAS No. |
1220910-90-6 |
---|---|
Molecular Formula |
C34H30F2N12O11P2 |
Molecular Weight |
882.631 |
IUPAC Name |
[[(5R)-3-[3-fluoro-4-[6-(2-methyltetrazol-5-yl)pyridin-3-yl]phenyl]-2-oxo-1,3-oxazolidin-5-yl]methoxy-hydroxyphosphoryl] [(5R)-3-[3-fluoro-4-[6-(2-methyltetrazol-5-yl)pyridin-3-yl]phenyl]-2-oxo-1,3-oxazolidin-5-yl]methyl hydrogen phosphate |
InChI |
InChI=1S/C34H30F2N12O11P2/c1-45-41-31(39-43-45)29-9-3-19(13-37-29)25-7-5-21(11-27(25)35)47-15-23(57-33(47)49)17-55-60(51,52)59-61(53,54)56-18-24-16-48(34(50)58-24)22-6-8-26(28(36)12-22)20-4-10-30(38-14-20)32-40-44-46(2)42-32/h3-14,23-24H,15-18H2,1-2H3,(H,51,52)(H,53,54)/t23-,24-/m1/s1 |
InChI Key |
DBBJYEOIIWGIRZ-DNQXCXABSA-N |
SMILES |
CN1N=C(N=N1)C2=NC=C(C=C2)C3=C(C=C(C=C3)N4CC(OC4=O)COP(=O)(O)OP(=O)(O)OCC5CN(C(=O)O5)C6=CC(=C(C=C6)C7=CN=C(C=C7)C8=NN(N=N8)C)F)F |
Synonyms |
P,P’-bis[[(5R)-3-[3-Fluoro-4-[6-(2-methyl-2H-tetrazol-5-yl)-3-pyridinyl]phenyl]-2-oxo-5-oxazolidinyl]methyl]diphosphoric Acid Ester |
Origin of Product |
United States |
Disclaimer and Information on In-Vitro Research Products
Please be aware that all articles and product information presented on BenchChem are intended solely for informational purposes. The products available for purchase on BenchChem are specifically designed for in-vitro studies, which are conducted outside of living organisms. In-vitro studies, derived from the Latin term "in glass," involve experiments performed in controlled laboratory settings using cells or tissues. It is important to note that these products are not categorized as medicines or drugs, and they have not received approval from the FDA for the prevention, treatment, or cure of any medical condition, ailment, or disease. We must emphasize that any form of bodily introduction of these products into humans or animals is strictly prohibited by law. It is essential to adhere to these guidelines to ensure compliance with legal and ethical standards in research and experimentation.