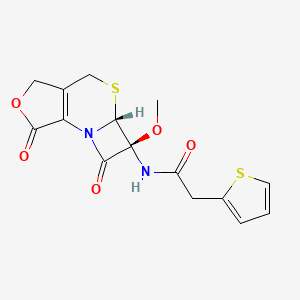
Decarbamylcefoxitin Lactone
Overview
Description
Decarbamylcefoxitin Lactone is a chemical compound identified by the CAS number 1422023-33-3. It is an impurity of Cefoxitin, a semi-synthetic antibiotic derived from Cephamycin C, known for its high resistance to β-lactamase inactivation . This compound is primarily used in analytical method development, method validation, and quality control applications for Abbreviated New Drug Applications and commercial production of Cefoxitin .
Mechanism of Action
Target of Action
Decarbamylcefoxitin Lactone, also known as N-[(4S,5R)-4-Methoxy-3,11-dioxo-10-oxa-6-thia-2-azatricyclo[6.3.0.02,5]undec-1(8)-en-4-yl]-2-thiophen-2-ylacetamide, is a semi-synthetic, broad-spectrum antibiotic . Its primary targets are bacterial penicillin-binding proteins (PBPs), which play a crucial role in the synthesis of peptidoglycan, a core component of the bacterial cell wall .
Mode of Action
This compound acts as a covalent inhibitor that interacts with its targets, the PBPs, and disrupts peptidoglycan synthesis . This disruption inhibits the growth of the offending microorganism and can even kill susceptible bacteria .
Biochemical Pathways
The compound’s action affects several biochemical pathways. One of the main pathways is the β-oxidation pathway, which is commonly used in the production of lactones . Other pathways include the ω-oxidation and the fungal polyketide pathway, which is relatively similar to the fatty acid synthesis pathway .
Pharmacokinetics
Similar compounds like cefoxitin have been studied, and their pharmacokinetic properties might provide some insights .
Result of Action
The bactericidal action of this compound results from the inhibition of cell wall synthesis . This leads to the death of the bacteria or inhibition of their growth . Bacteria can achieve resistance to β-lactams in several ways, including the production of serine β-lactamase enzymes .
Action Environment
The action, efficacy, and stability of this compound can be influenced by various environmental factors. For instance, the concentration of the antibiotic at the site of infection must be sufficient to inhibit the growth of the offending microorganism . If host defenses are impaired, antibiotic-mediated killing may be required to eradicate the infection . Furthermore, the compound’s equilibrium behavior can be influenced by the copolymerization with more thermodynamically favored monomers .
Biochemical Analysis
Biochemical Properties
Decarbamylcefoxitin Lactone plays a significant role in biochemical reactions, particularly due to its β-lactone structure. This compound interacts with enzymes such as β-lactamases, which are responsible for hydrolyzing the β-lactam ring, rendering antibiotics ineffective . The interaction between this compound and β-lactamases is crucial as it helps in understanding the resistance mechanisms of bacteria against β-lactam antibiotics. Additionally, this compound may interact with other proteins and biomolecules involved in cell wall synthesis and degradation.
Cellular Effects
This compound influences various cellular processes, including cell signaling pathways, gene expression, and cellular metabolism. This compound can affect the function of bacterial cells by inhibiting the synthesis of their cell walls, leading to cell lysis and death . In mammalian cells, this compound may impact cell signaling pathways such as the NF-κB pathway, which is involved in inflammation and immune responses
Molecular Mechanism
The molecular mechanism of this compound involves its interaction with β-lactamases and other enzymes involved in cell wall synthesis. The compound binds to the active site of β-lactamases, inhibiting their activity and preventing the hydrolysis of β-lactam antibiotics . This inhibition leads to the accumulation of β-lactam antibiotics, which can then exert their bactericidal effects. Additionally, this compound may interact with other biomolecules, leading to changes in gene expression and enzyme activity.
Temporal Effects in Laboratory Settings
In laboratory settings, the effects of this compound can change over time. The compound’s stability and degradation are important factors that influence its long-term effects on cellular function. Studies have shown that this compound is relatively stable under certain conditions, but it can degrade over time, leading to a decrease in its efficacy . Long-term exposure to this compound may result in adaptive changes in bacterial cells, such as the upregulation of resistance genes.
Dosage Effects in Animal Models
The effects of this compound vary with different dosages in animal models. At low doses, the compound may exhibit minimal toxicity and effectively inhibit bacterial growth . At higher doses, this compound may cause adverse effects, such as toxicity to mammalian cells and disruption of normal cellular functions. Threshold effects have been observed, where a certain dosage is required to achieve the desired antibacterial effects without causing significant toxicity.
Metabolic Pathways
This compound is involved in various metabolic pathways, including those related to the synthesis and degradation of cell wall components. The compound interacts with enzymes such as β-lactamases and other proteins involved in the β-lactam antibiotic resistance pathway . These interactions can affect metabolic flux and metabolite levels, leading to changes in cellular metabolism and the development of antibiotic resistance.
Transport and Distribution
The transport and distribution of this compound within cells and tissues are influenced by various factors, including its interaction with transporters and binding proteins . The compound can be transported across cell membranes through passive diffusion or active transport mechanisms. Once inside the cell, this compound may accumulate in specific compartments, such as the cytoplasm or cell wall, where it can exert its effects.
Subcellular Localization
This compound is localized in specific subcellular compartments, which can influence its activity and function. The compound may be directed to the cell wall or cytoplasm through targeting signals or post-translational modifications . This localization is crucial for its interaction with β-lactamases and other enzymes involved in cell wall synthesis and degradation. Understanding the subcellular localization of this compound can provide insights into its mechanism of action and potential therapeutic applications.
Preparation Methods
The synthesis of lactones, including Decarbamylcefoxitin Lactone, can be achieved through various methods. One common approach involves the dehydrogenation of unprotected or partially protected alditols and aldoses catalyzed by a transition metal complex in the presence of a hydrogen acceptor . Another method includes the selective γ-C(sp3)–H activation of aliphatic carboxylic acids in the presence of a palladium catalyst, which provides six or seven-membered lactones depending on the olefin partner .
Chemical Reactions Analysis
Decarbamylcefoxitin Lactone undergoes various chemical reactions, including:
Oxidation: Lactones can be oxidized to form carboxylic acids or other oxidized derivatives.
Reduction: Reduction of lactones typically yields diols.
Substitution: Lactones can undergo nucleophilic substitution reactions, particularly at the carbonyl carbon. Common reagents used in these reactions include oxidizing agents like potassium permanganate, reducing agents like lithium aluminum hydride, and nucleophiles such as amines and alcohols
Scientific Research Applications
Decarbamylcefoxitin Lactone has several scientific research applications:
Comparison with Similar Compounds
Properties
IUPAC Name |
N-[(4S,5R)-4-methoxy-3,11-dioxo-10-oxa-6-thia-2-azatricyclo[6.3.0.02,5]undec-1(8)-en-4-yl]-2-thiophen-2-ylacetamide | |
---|---|---|
Source | PubChem | |
URL | https://pubchem.ncbi.nlm.nih.gov | |
Description | Data deposited in or computed by PubChem | |
InChI |
InChI=1S/C15H14N2O5S2/c1-21-15(16-10(18)5-9-3-2-4-23-9)13(20)17-11-8(6-22-12(11)19)7-24-14(15)17/h2-4,14H,5-7H2,1H3,(H,16,18)/t14-,15+/m1/s1 | |
Source | PubChem | |
URL | https://pubchem.ncbi.nlm.nih.gov | |
Description | Data deposited in or computed by PubChem | |
InChI Key |
ZNHLCKJVGCWLCR-CABCVRRESA-N | |
Source | PubChem | |
URL | https://pubchem.ncbi.nlm.nih.gov | |
Description | Data deposited in or computed by PubChem | |
Canonical SMILES |
COC1(C2N(C1=O)C3=C(COC3=O)CS2)NC(=O)CC4=CC=CS4 | |
Source | PubChem | |
URL | https://pubchem.ncbi.nlm.nih.gov | |
Description | Data deposited in or computed by PubChem | |
Isomeric SMILES |
CO[C@@]1([C@@H]2N(C1=O)C3=C(COC3=O)CS2)NC(=O)CC4=CC=CS4 | |
Source | PubChem | |
URL | https://pubchem.ncbi.nlm.nih.gov | |
Description | Data deposited in or computed by PubChem | |
Molecular Formula |
C15H14N2O5S2 | |
Source | PubChem | |
URL | https://pubchem.ncbi.nlm.nih.gov | |
Description | Data deposited in or computed by PubChem | |
Molecular Weight |
366.4 g/mol | |
Source | PubChem | |
URL | https://pubchem.ncbi.nlm.nih.gov | |
Description | Data deposited in or computed by PubChem | |
CAS No. |
1422023-33-3 | |
Record name | Cefoxitin lactone | |
Source | FDA Global Substance Registration System (GSRS) | |
URL | https://gsrs.ncats.nih.gov/ginas/app/beta/substances/MAZ3H38FEJ | |
Description | The FDA Global Substance Registration System (GSRS) enables the efficient and accurate exchange of information on what substances are in regulated products. Instead of relying on names, which vary across regulatory domains, countries, and regions, the GSRS knowledge base makes it possible for substances to be defined by standardized, scientific descriptions. | |
Explanation | Unless otherwise noted, the contents of the FDA website (www.fda.gov), both text and graphics, are not copyrighted. They are in the public domain and may be republished, reprinted and otherwise used freely by anyone without the need to obtain permission from FDA. Credit to the U.S. Food and Drug Administration as the source is appreciated but not required. | |
Disclaimer and Information on In-Vitro Research Products
Please be aware that all articles and product information presented on BenchChem are intended solely for informational purposes. The products available for purchase on BenchChem are specifically designed for in-vitro studies, which are conducted outside of living organisms. In-vitro studies, derived from the Latin term "in glass," involve experiments performed in controlled laboratory settings using cells or tissues. It is important to note that these products are not categorized as medicines or drugs, and they have not received approval from the FDA for the prevention, treatment, or cure of any medical condition, ailment, or disease. We must emphasize that any form of bodily introduction of these products into humans or animals is strictly prohibited by law. It is essential to adhere to these guidelines to ensure compliance with legal and ethical standards in research and experimentation.