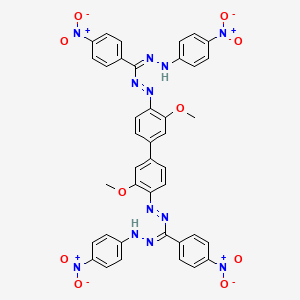
TNBT Diformazan
- Click on QUICK INQUIRY to receive a quote from our team of experts.
- With the quality product at a COMPETITIVE price, you can focus more on your research.
Overview
Description
TNBT Diformazan: is a chemical compound known for its intense color and its use in various biochemical assays. It is derived from the reduction of nitro-blue tetrazolium (NBT) and is often used as a histochemical marker. The compound is characterized by its deep yellow-red color and is commonly used in biological and chemical research.
Preparation Methods
Synthetic Routes and Reaction Conditions: The synthesis of TNBT Diformazan typically involves the reduction of nitro-blue tetrazolium (NBT) in the presence of reducing agents such as NADPH. The reaction conditions often include the use of aldehyde-treated tissues, where the catalytic activity of nitric oxide synthase (NOS) promotes the reduction of NBT to diformazan .
Industrial Production Methods:
Chemical Reactions Analysis
Types of Reactions: TNBT Diformazan primarily undergoes reduction reactions. The reduction of NBT to this compound is facilitated by the presence of reducing agents such as NADPH and S-nitrosothiols .
Common Reagents and Conditions:
Reducing Agents: NADPH, S-nitrosothiols
Reaction Conditions: Presence of nitric oxide synthase (NOS), aldehyde-treated tissues
Major Products: The major product of the reduction of NBT is this compound, which is characterized by its deep yellow-red color .
Scientific Research Applications
Chemistry: TNBT Diformazan is used as a chromogenic agent in various chemical assays due to its intense color. It is also used in the study of redox reactions and the behavior of reducing agents .
Biology: In biological research, this compound is used as a histochemical marker to detect the presence of nitric oxide synthase (NOS) in tissues. It is also used in cell viability assays and to study the distribution of S-nitrosothiols in biological tissues .
Medicine: this compound is used in medical research to study the effects of nitric oxide and related compounds in various physiological and pathological processes .
Industry: In industrial applications, this compound is used in the production of dyes and pigments due to its intense color. It is also used in the development of diagnostic assays and biochemical tests .
Mechanism of Action
The mechanism of action of TNBT Diformazan involves its reduction from nitro-blue tetrazolium (NBT) in the presence of reducing agents such as NADPH and S-nitrosothiols. The catalytic activity of nitric oxide synthase (NOS) promotes the reduction of NBT to diformazan, which is then visualized as a deep yellow-red color in histochemical assays .
Comparison with Similar Compounds
Nitro-blue tetrazolium (NBT): The precursor to TNBT Diformazan, used in similar biochemical assays.
Tetranitroblue tetrazolium (TNBT): Another tetrazolium compound used in histochemical staining.
Uniqueness: this compound is unique due to its intense color and its specific use in detecting nitric oxide synthase (NOS) activity in biological tissues. Its ability to form a deep yellow-red color upon reduction makes it a valuable tool in various biochemical and histochemical assays .
Properties
CAS No. |
19333-63-2 |
---|---|
Molecular Formula |
C40H30N12O10 |
Molecular Weight |
838.7 g/mol |
IUPAC Name |
N-[2-methoxy-4-[3-methoxy-4-[[(E)-N-(4-nitroanilino)-C-(4-nitrophenyl)carbonimidoyl]diazenyl]phenyl]phenyl]imino-4-nitro-N'-(4-nitroanilino)benzenecarboximidamide |
InChI |
InChI=1S/C40H30N12O10/c1-61-37-23-27(7-21-35(37)43-47-39(25-3-13-31(14-4-25)49(53)54)45-41-29-9-17-33(18-10-29)51(57)58)28-8-22-36(38(24-28)62-2)44-48-40(26-5-15-32(16-6-26)50(55)56)46-42-30-11-19-34(20-12-30)52(59)60/h3-24,41-42H,1-2H3/b45-39+,46-40+,47-43?,48-44? |
InChI Key |
SDKSRZQMARNUEW-SAORWRKWSA-N |
Isomeric SMILES |
COC1=C(C=CC(=C1)C2=CC(=C(C=C2)N=N/C(=N/NC3=CC=C(C=C3)[N+](=O)[O-])/C4=CC=C(C=C4)[N+](=O)[O-])OC)N=N/C(=N/NC5=CC=C(C=C5)[N+](=O)[O-])/C6=CC=C(C=C6)[N+](=O)[O-] |
SMILES |
COC1=C(C=CC(=C1)C2=CC(=C(C=C2)N=NC(=NNC3=CC=C(C=C3)[N+](=O)[O-])C4=CC=C(C=C4)[N+](=O)[O-])OC)N=NC(=NNC5=CC=C(C=C5)[N+](=O)[O-])C6=CC=C(C=C6)[N+](=O)[O-] |
Canonical SMILES |
COC1=C(C=CC(=C1)C2=CC(=C(C=C2)N=NC(=NNC3=CC=C(C=C3)[N+](=O)[O-])C4=CC=C(C=C4)[N+](=O)[O-])OC)N=NC(=NNC5=CC=C(C=C5)[N+](=O)[O-])C6=CC=C(C=C6)[N+](=O)[O-] |
Origin of Product |
United States |
Disclaimer and Information on In-Vitro Research Products
Please be aware that all articles and product information presented on BenchChem are intended solely for informational purposes. The products available for purchase on BenchChem are specifically designed for in-vitro studies, which are conducted outside of living organisms. In-vitro studies, derived from the Latin term "in glass," involve experiments performed in controlled laboratory settings using cells or tissues. It is important to note that these products are not categorized as medicines or drugs, and they have not received approval from the FDA for the prevention, treatment, or cure of any medical condition, ailment, or disease. We must emphasize that any form of bodily introduction of these products into humans or animals is strictly prohibited by law. It is essential to adhere to these guidelines to ensure compliance with legal and ethical standards in research and experimentation.