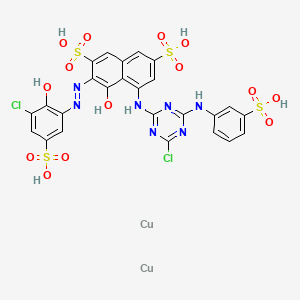
Reactive violet 1
- Click on QUICK INQUIRY to receive a quote from our team of experts.
- With the quality product at a COMPETITIVE price, you can focus more on your research.
Overview
Description
Reactive Violet 1 is a synthetic dye belonging to the class of reactive dyes. These dyes are known for their ability to form covalent bonds with substrates, making them highly effective for dyeing textiles, particularly cotton fabrics. This compound is characterized by its vibrant violet color and is widely used in various industrial applications due to its excellent colorfastness and stability.
Preparation Methods
Synthetic Routes and Reaction Conditions: The synthesis of Reactive Violet 1 typically involves the reaction of an aromatic amine with a diazonium salt, followed by coupling with a suitable coupling component. The reaction conditions often include maintaining a specific pH and temperature to ensure optimal yield and purity of the dye.
Industrial Production Methods: Industrial production of this compound involves large-scale chemical synthesis using automated reactors. The process includes the precise control of reaction parameters such as temperature, pH, and reactant concentrations. The final product is then purified through filtration, crystallization, and drying to obtain the dye in its pure form.
Chemical Reactions Analysis
Types of Reactions: Reactive Violet 1 undergoes various chemical reactions, including:
Oxidation: The dye can be oxidized under specific conditions, leading to the formation of different oxidation products.
Reduction: this compound can be reduced to its corresponding amine derivatives.
Substitution: The dye can participate in substitution reactions, where functional groups on the dye molecule are replaced by other groups.
Common Reagents and Conditions:
Oxidation: Common oxidizing agents include hydrogen peroxide and potassium permanganate.
Reduction: Reducing agents such as sodium borohydride and zinc dust are often used.
Substitution: Substitution reactions typically involve reagents like halogens or nucleophiles under controlled pH and temperature conditions.
Major Products Formed: The major products formed from these reactions depend on the specific conditions and reagents used. For example, oxidation may yield quinonoid structures, while reduction can produce amine derivatives.
Scientific Research Applications
Reactive Violet 1 has a wide range of applications in scientific research, including:
Chemistry: Used as a model compound for studying dye degradation and photocatalytic processes.
Biology: Employed in staining techniques for visualizing cellular components.
Medicine: Investigated for its potential use in photodynamic therapy and as a diagnostic tool.
Industry: Widely used in textile dyeing, paper printing, and as a colorant in various products.
Mechanism of Action
The mechanism of action of Reactive Violet 1 involves the formation of covalent bonds with the substrate, typically through nucleophilic substitution reactions. The dye molecules react with hydroxyl or amino groups on the substrate, forming stable covalent bonds. This results in the dye being permanently attached to the substrate, providing excellent colorfastness and resistance to washing and light.
Comparison with Similar Compounds
- Reactive Green 19
- Reactive Orange 84
- Reactive Yellow 81
Comparison: Reactive Violet 1 is unique due to its specific molecular structure, which imparts its characteristic violet color. Compared to other reactive dyes, it offers superior colorfastness and stability, making it highly desirable for industrial applications. While other reactive dyes like Reactive Green 19 and Reactive Orange 84 also form covalent bonds with substrates, their color properties and specific applications may vary.
Properties
IUPAC Name |
3-[(3-chloro-2-hydroxy-5-sulfophenyl)diazenyl]-5-[[4-chloro-6-(3-sulfoanilino)-1,3,5-triazin-2-yl]amino]-4-hydroxynaphthalene-2,7-disulfonic acid;copper |
Source
|
---|---|---|
Source | PubChem | |
URL | https://pubchem.ncbi.nlm.nih.gov | |
Description | Data deposited in or computed by PubChem | |
InChI |
InChI=1S/C25H17Cl2N7O14S4.2Cu/c26-15-7-14(51(43,44)45)9-17(21(15)35)33-34-20-18(52(46,47)48)5-10-4-13(50(40,41)42)8-16(19(10)22(20)36)29-25-31-23(27)30-24(32-25)28-11-2-1-3-12(6-11)49(37,38)39;;/h1-9,35-36H,(H,37,38,39)(H,40,41,42)(H,43,44,45)(H,46,47,48)(H2,28,29,30,31,32);; |
Source
|
Source | PubChem | |
URL | https://pubchem.ncbi.nlm.nih.gov | |
Description | Data deposited in or computed by PubChem | |
InChI Key |
DJIZTAVUUUPKQR-UHFFFAOYSA-N |
Source
|
Source | PubChem | |
URL | https://pubchem.ncbi.nlm.nih.gov | |
Description | Data deposited in or computed by PubChem | |
Canonical SMILES |
C1=CC(=CC(=C1)S(=O)(=O)O)NC2=NC(=NC(=N2)Cl)NC3=C4C(=CC(=C3)S(=O)(=O)O)C=C(C(=C4O)N=NC5=C(C(=CC(=C5)S(=O)(=O)O)Cl)O)S(=O)(=O)O.[Cu].[Cu] |
Source
|
Source | PubChem | |
URL | https://pubchem.ncbi.nlm.nih.gov | |
Description | Data deposited in or computed by PubChem | |
Molecular Formula |
C25H17Cl2Cu2N7O14S4 |
Source
|
Source | PubChem | |
URL | https://pubchem.ncbi.nlm.nih.gov | |
Description | Data deposited in or computed by PubChem | |
Molecular Weight |
965.7 g/mol |
Source
|
Source | PubChem | |
URL | https://pubchem.ncbi.nlm.nih.gov | |
Description | Data deposited in or computed by PubChem | |
CAS No. |
12239-45-1 |
Source
|
Record name | C.I. Reactive Violet 1 | |
Source | European Chemicals Agency (ECHA) | |
URL | https://echa.europa.eu/information-on-chemicals | |
Description | The European Chemicals Agency (ECHA) is an agency of the European Union which is the driving force among regulatory authorities in implementing the EU's groundbreaking chemicals legislation for the benefit of human health and the environment as well as for innovation and competitiveness. | |
Explanation | Use of the information, documents and data from the ECHA website is subject to the terms and conditions of this Legal Notice, and subject to other binding limitations provided for under applicable law, the information, documents and data made available on the ECHA website may be reproduced, distributed and/or used, totally or in part, for non-commercial purposes provided that ECHA is acknowledged as the source: "Source: European Chemicals Agency, http://echa.europa.eu/". Such acknowledgement must be included in each copy of the material. ECHA permits and encourages organisations and individuals to create links to the ECHA website under the following cumulative conditions: Links can only be made to webpages that provide a link to the Legal Notice page. | |
Disclaimer and Information on In-Vitro Research Products
Please be aware that all articles and product information presented on BenchChem are intended solely for informational purposes. The products available for purchase on BenchChem are specifically designed for in-vitro studies, which are conducted outside of living organisms. In-vitro studies, derived from the Latin term "in glass," involve experiments performed in controlled laboratory settings using cells or tissues. It is important to note that these products are not categorized as medicines or drugs, and they have not received approval from the FDA for the prevention, treatment, or cure of any medical condition, ailment, or disease. We must emphasize that any form of bodily introduction of these products into humans or animals is strictly prohibited by law. It is essential to adhere to these guidelines to ensure compliance with legal and ethical standards in research and experimentation.