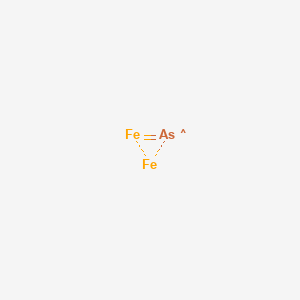
Iron arsenide
- Click on QUICK INQUIRY to receive a quote from our team of experts.
- With the quality product at a COMPETITIVE price, you can focus more on your research.
Overview
Description
Iron arsenide is a compound of arsenic with a less electronegative element, iron . Many metals form binary compounds containing arsenic, and these are called arsenides . They exist with many stoichiometries, and in this respect arsenides are similar to phosphides . This compound is a crystalline solid with a slight yellow or brown color .
Synthesis Analysis
This compound clusters can be synthesized by the reaction between K5As4 and a low valent FeII complex, [K(THF)Fe(OtBu)3]2 (where THF is tetrahydrofuran), in the presence of a cryptand ([2.2.2.]crypt) or 18-crown-6, occurs at 60 °C . A new iron-based superconductor (Ca,Pr)FeAs2 was discovered .Molecular Structure Analysis
This compound compounds contain a triangular Fe3 core . The structure of this compound is complex with unusually short Na–Na distances of 328–330 pm which are shorter than in sodium metal . This short distance indicates the complex bonding in these simple phases .Chemical Reactions Analysis
This compound can break down under high-temperature conditions . The decomposition of iron arsenides is directly associated with the effectiveness of As removal from arsenic-bearing gold concentrates .Physical And Chemical Properties Analysis
This compound is a crystalline powder with a slight yellow or brown color . It is insoluble in water and has a relatively high melting point, which makes it stable under normal environmental conditions .Scientific Research Applications
Superconductivity : Iron arsenide compounds, such as BaFe2As2, exhibit superconductivity. The spin-density-wave (SDW) anomaly in these compounds, similar to that in LaFeAsO, is crucial to their superconducting properties. BaFe2As2, for example, undergoes a structural and magnetic phase transition accompanied by strong anomalies in specific heat, electrical resistance, and magnetic susceptibility, serving as a parent compound for oxygen-free this compound superconductors (Rotter, Tegel, & Johrendt, 2008). Additionally, superconductivity at 38 K has been observed in BaFe2As2 by hole doping through partial substitution of barium with potassium (Rotter, Tegel, & Johrendt, 2008).
Water Treatment : Nano-sized zero-valent iron is effective in removing arsenic from drinking water. When supported on activated carbon, it shows fast adsorption kinetics and high adsorption capacity for arsenite and arsenate, making it suitable for water treatment systems (Zhu, Jia, Wu, & Wang, 2009).
Magnetic Excitation in Superconductors : In the high-temperature superconductor Ba0.6K0.4Fe2As2, inelastic neutron scattering observations reveal a magnetic resonance in the superconducting phase, indicating unconventional symmetry in the superconducting energy gap. This discovery is crucial for understanding the nature of electron pairing in this compound superconductors (Christianson et al., 2008).
Magnetic and Structural Phase Transitions : The iron arsenides experience magnetic and structural phase transitions that need to be suppressed for superconductivity to emerge. The close proximity of these transition temperatures suggests a correlation between these phases, possibly with magnetic fluctuations driving structural transitions (Fernandes, Böhmer, Meingast, & Schmalian, 2013).
Arsenic Remediation : Zero-valent iron (Fe0) shows potential for removing arsenic from groundwater and drinking water, with reactions resulting in high uptake of both arsenate and arsenite. The corrosion products of Fe0, including various iron oxides, indicate oxidation of Fe(II) as an intermediate step in the corrosion process, beneficial for arsenic removal (Manning, Hunt, Amrhein, & Yarmoff, 2002).
Mechanism of Action
Target of Action
Iron arsenide, also known as MFCD00151533, is primarily used in the field of semiconductors , superconductors , and photo optic applications . It is also a key component in the extraction of gold from double refractory gold-arsenic-bearing carbonaceous ores .
Mode of Action
This compound interacts with its targets through a series of complex reactions. For instance, in the context of gold extraction, this compound plays a crucial role in locking arsenic into this compound phases under reducing atmospheres in the presence of an iron-rich slag . This process is essential for the effective removal of arsenic from arsenic-bearing gold concentrates .
Biochemical Pathways
Arsenic, a component of this compound, is known to undergo various biotransformation processes such as uptake, accumulation, reduction, oxidation, methylation, and demethylation . These processes involve a certain set of genes and proteins
Pharmacokinetics
It is known that this compound is insoluble in water , which could impact its bioavailability and distribution in the body
Result of Action
Arsenic, a component of this compound, is known to have toxic effects on cells
Action Environment
The action of this compound can be influenced by various environmental factors. For instance, the presence of oxalate on the adsorbent surface plays an important role in the recycling of Fe (II)/Fe (III) to minimize the arsenic concentration . Additionally, microbial-mediated redox of iron has an important influence on the environmental behavior of arsenic .
Safety and Hazards
Future Directions
Iron arsenide monolayers are known as a key component for building iron-based superconductors . They are predicted to be a highly stable and multiferroic material with coexisting ferroelasticity and antiferromagnetism . The superior magnetic and electronic properties of the FeAs monolayers make them promising candidates for spintronics applications .
Biochemical Analysis
Biochemical Properties
Iron arsenide plays a significant role in biochemical reactions . It interacts with various enzymes, proteins, and other biomolecules. The nature of these interactions is complex and multifaceted, involving various biochemical pathways .
Cellular Effects
This compound has profound effects on various types of cells and cellular processes . It influences cell function, including impacts on cell signaling pathways, gene expression, and cellular metabolism .
Molecular Mechanism
The mechanism of action of this compound is intricate. It exerts its effects at the molecular level, including binding interactions with biomolecules, enzyme inhibition or activation, and changes in gene expression .
Temporal Effects in Laboratory Settings
In laboratory settings, the effects of this compound change over time. Information on the product’s stability, degradation, and any long-term effects on cellular function observed in in vitro or in vivo studies is continually being updated .
Dosage Effects in Animal Models
The effects of this compound vary with different dosages in animal models . Studies have observed threshold effects, as well as toxic or adverse effects at high doses .
Metabolic Pathways
This compound is involved in various metabolic pathways. It interacts with several enzymes or cofactors, and can affect metabolic flux or metabolite levels .
Transport and Distribution
This compound is transported and distributed within cells and tissues in a specific manner . It interacts with certain transporters or binding proteins, and can affect its localization or accumulation .
Subcellular Localization
The subcellular localization of this compound and its effects on its activity or function are areas of active research . It could include any targeting signals or post-translational modifications that direct it to specific compartments or organelles .
properties
{ "Design of the Synthesis Pathway": "Iron arsenide can be synthesized using solid-state reaction method or chemical vapor transport method. In the solid-state reaction method, iron and arsenic are reacted at high temperatures to form iron arsenide. In the chemical vapor transport method, a gaseous arsenic compound is reacted with iron at high temperatures to form iron arsenide.", "Starting Materials": ["Iron", "Arsenic"], "Reaction": ["1. Weigh the appropriate amount of iron and arsenic.", "2. Grind the iron and arsenic together to form a homogeneous mixture.", "3. Place the mixture in a ceramic crucible and heat it in a furnace under an inert atmosphere.", "4. Heat the mixture to a temperature of about 800-1000°C and hold it at this temperature for several hours.", "5. Cool the crucible and remove the synthesized iron arsenide." ] } | |
CAS RN |
12005-88-8 |
Molecular Formula |
AsFe2 |
Molecular Weight |
186.61 g/mol |
InChI |
InChI=1S/As.2Fe |
InChI Key |
FDKAYGUKROYPRO-UHFFFAOYSA-N |
SMILES |
[Fe].[Fe]=[As] |
Canonical SMILES |
[Fe].[Fe]=[As] |
Origin of Product |
United States |
Q & A
Q1: What is the basic structural motif of iron arsenide superconductors?
A1: The key structural element in this compound superconductors is a square lattice of iron atoms, with pnictogen or chalcogen atoms (like arsenic, phosphorus, selenium, or tellurium) situated above and below the iron plane in a tetrahedral coordination. [] This contrasts with the oxygen octahedra found in cuprate superconductors.
Q2: How does the FeAs4 tetrahedron geometry influence superconductivity in iron arsenides?
A2: Research suggests a correlation between the As-Fe-As bond angles in the FeAs4 tetrahedra and the superconducting transition temperature (Tc). More regular, less distorted tetrahedra generally correspond to higher Tc values. [] For instance, LiFeAs exhibits compressed FeAs4 tetrahedra in the basal plane compared to other this compound superconductors, and applying pressure further distorts these tetrahedra while decreasing Tc. []
Q3: Can you elaborate on the structural transitions observed in iron arsenides?
A4: Many iron arsenides undergo a structural transition from a tetragonal to an orthorhombic phase upon cooling. This is often followed by the emergence of antiferromagnetic ordering at even lower temperatures. [, , ] For instance, LaFeAsO undergoes this tetragonal-orthorhombic transition around 160 K, followed by antiferromagnetic ordering near 145 K. []
Q4: How does doping affect the structure and properties of iron arsenides?
A5: Doping plays a crucial role in inducing superconductivity in iron arsenides. For example, BaFe2As2, a non-superconducting parent compound, becomes superconducting upon hole doping with potassium (Ba1-xKxFe2As2). [] This doping suppresses the SDW anomaly present in the parent compound and gives rise to superconductivity. Similarly, electron doping in BaFe2As2, achieved through cobalt or nickel substitution at the iron sites, also induces superconductivity. []
Q5: What is the role of magnetism in this compound superconductors?
A6: The interplay between magnetism and superconductivity is a defining characteristic of iron arsenides. Most undoped parent compounds exhibit antiferromagnetic ordering at low temperatures. [, ] Superconductivity emerges when this magnetic order is suppressed, typically through doping or the application of pressure. [, , ]
Q6: How does the electronic structure of iron arsenides differ from cuprate superconductors?
A7: Unlike cuprate superconductors, which are Mott insulators in their undoped state, iron arsenides are poor metals. [] Additionally, iron arsenides have multiple bands crossing the Fermi level, leading to a more complex electronic structure compared to cuprates, which primarily involve a single band. []
Q7: What is the significance of the "resonance" observed in inelastic neutron scattering experiments on iron arsenides?
A8: The "resonance," a peak in the spin excitation spectrum observed in materials like BaFe1.9Ni0.1As2, provides strong evidence for an unconventional pairing mechanism in this compound superconductors. [] The resonance energy is closely tied to the superconducting gap energy, suggesting that spin fluctuations play a vital role in mediating electron pairing. []
Q8: What experimental techniques are used to study the electronic structure of iron arsenides?
A9: Various techniques are employed to probe the electronic structure, including angle-resolved photoemission spectroscopy (ARPES), [] inelastic neutron scattering, [, , ] and optical spectroscopy. [, ] These techniques help researchers understand the Fermi surface topology, the superconducting gap structure, and the nature of electronic correlations in these materials.
Q9: What synthesis methods are commonly used to prepare this compound superconductors?
A10: High-temperature solid-state reactions are frequently employed to synthesize iron arsenides. [, , ] This often involves sealing precursor materials in evacuated ampoules and subjecting them to controlled heating profiles. High-pressure synthesis methods, sometimes coupled with rapid quenching processes, are also utilized, particularly for synthesizing compounds with heavier rare-earth elements like HoFeAsO1-δ. []
Q10: Are there any challenges associated with synthesizing these materials?
A11: Achieving the desired stoichiometry and phase purity can be challenging. For instance, in the Sr1-xNaxFe2As2 system, the sodium content achievable through conventional solid-state synthesis is limited (x = 0.4). [] Higher sodium concentrations lead to the formation of impurity phases like elemental iron. []
Q11: What are the potential applications of this compound superconductors?
A11: While still in the research phase, this compound superconductors hold promise for various applications:
Disclaimer and Information on In-Vitro Research Products
Please be aware that all articles and product information presented on BenchChem are intended solely for informational purposes. The products available for purchase on BenchChem are specifically designed for in-vitro studies, which are conducted outside of living organisms. In-vitro studies, derived from the Latin term "in glass," involve experiments performed in controlled laboratory settings using cells or tissues. It is important to note that these products are not categorized as medicines or drugs, and they have not received approval from the FDA for the prevention, treatment, or cure of any medical condition, ailment, or disease. We must emphasize that any form of bodily introduction of these products into humans or animals is strictly prohibited by law. It is essential to adhere to these guidelines to ensure compliance with legal and ethical standards in research and experimentation.