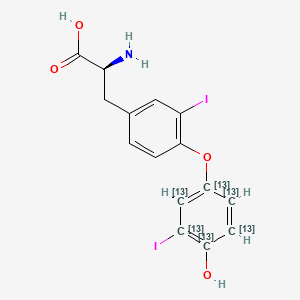
3, 3'-Diiodo-L-thyronine-(phenoxy-13C6) (T2)
Overview
Description
3, 3'-Diiodo-L-thyronine-(phenoxy-13C6) (T2) is a complex organic compound characterized by its unique structure, which includes multiple iodine atoms and a cyclohexa-1,3,5-trien-1-yl group
Mechanism of Action
3,3’-Diiodo-L-thyronine-13C6: is an endogenous metabolite of thyroid hormone. Its primary targets include thyroid receptors (TRs) within cells. These TRs play a crucial role in regulating gene transcription via thyroid response elements (TREs) . The binding of 3,3’-T2-13C6 to TRs initiates a cascade of events that influence cellular processes.
Mode of Action:
The classical mechanism of thyroid hormone action involves a two-step process:
- Interaction : An interaction occurs between a chemically reactive group on the hormone and a complementary component on the receptor. This interaction leads to chemical or conformational changes in the receptor, ultimately affecting gene transcription .
Action Environment:
Environmental factors, such as temperature, pH, and coexisting molecules, can influence 3,3’-T2-13C6 efficacy and stability. For instance, variations in pH may affect its binding affinity to TRs.
: Lorenzini L, et al. Assay of Endogenous 3,5-diiodo-L-thyronine (3,5-T2) and 3,3’-diiodo-L-thyronine (3,3’-T2) in Human Serum: A Feasibility Study. Front Endocrinol (Lausanne). 2019 Feb 19;10:88. : Lanni A, et al. Rapid stimulation in vitro of rat liver cytochrome oxidase activity by 3,5-diiodo-L-thyronine and by 3,3’-diiodo-L-thyronine. Mol Cell Endocrinol. 1994 Feb 99(1):89-94.
Biochemical Analysis
Biochemical Properties
3,3’-Diiodo-L-thyronine-13C6 plays a crucial role in biochemical reactions. It interacts with various enzymes, proteins, and other biomolecules. The compound significantly enhances the activity of the enzyme COX . The nature of these interactions is complex and involves multiple biochemical pathways.
Cellular Effects
The effects of 3,3’-Diiodo-L-thyronine-13C6 on various types of cells and cellular processes are profound. It influences cell function by impacting cell signaling pathways, gene expression, and cellular metabolism
Molecular Mechanism
The molecular mechanism of action of 3,3’-Diiodo-L-thyronine-13C6 involves its binding interactions with biomolecules, enzyme inhibition or activation, and changes in gene expression
Preparation Methods
Synthetic Routes and Reaction Conditions
The synthesis of 3, 3'-Diiodo-L-thyronine-(phenoxy-13C6) (T2) typically involves multiple steps, including the iodination of phenolic compounds and the coupling of amino acids. The reaction conditions often require the use of strong oxidizing agents and specific catalysts to ensure the correct placement of iodine atoms and the formation of the desired cyclohexa-1,3,5-trien-1-yl group.
Industrial Production Methods
Industrial production methods for this compound are likely to involve large-scale synthesis techniques, including continuous flow reactors and automated synthesis systems. These methods ensure high yield and purity of the final product, which is essential for its use in scientific research and potential therapeutic applications.
Chemical Reactions Analysis
Types of Reactions
3, 3'-Diiodo-L-thyronine-(phenoxy-13C6) (T2) undergoes various types of chemical reactions, including:
Oxidation: The compound can be oxidized to form quinones and other oxidized derivatives.
Reduction: Reduction reactions can remove iodine atoms or reduce double bonds within the cyclohexa-1,3,5-trien-1-yl group.
Substitution: Halogen atoms, such as iodine, can be substituted with other functional groups using nucleophilic substitution reactions.
Common Reagents and Conditions
Common reagents used in these reactions include:
Oxidizing agents: Potassium permanganate (KMnO4), hydrogen peroxide (H2O2)
Reducing agents: Sodium borohydride (NaBH4), lithium aluminum hydride (LiAlH4)
Nucleophiles: Sodium azide (NaN3), thiols (R-SH)
Major Products
The major products formed from these reactions depend on the specific conditions and reagents used. For example, oxidation may yield quinones, while reduction can produce deiodinated or hydrogenated derivatives.
Scientific Research Applications
3, 3'-Diiodo-L-thyronine-(phenoxy-13C6) (T2) has a wide range of scientific research applications, including:
Chemistry: Used as a precursor in the synthesis of complex organic molecules and as a reagent in various chemical reactions.
Biology: Studied for its potential effects on cellular processes and as a tool for probing biological pathways.
Medicine: Investigated for its potential therapeutic properties, including antimicrobial and anticancer activities.
Industry: Utilized in the development of new materials and as a component in various industrial processes.
Comparison with Similar Compounds
Similar Compounds
- (2S)-2-Amino-3-[4-(4-hydroxy-3-iodophenyl)oxy-3-iodophenyl]propanoic acid
- (2S)-2-Amino-3-[4-(4-hydroxy-3-bromophenyl)oxy-3-iodophenyl]propanoic acid
- (2S)-2-Amino-3-[4-(4-hydroxy-3-chlorophenyl)oxy-3-iodophenyl]propanoic acid
Uniqueness
The uniqueness of 3, 3'-Diiodo-L-thyronine-(phenoxy-13C6) (T2) lies in its incorporation of multiple iodine atoms and the cyclohexa-1,3,5-trien-1-yl group. These features confer specific chemical reactivity and biological activity, distinguishing it from other similar compounds.
Biological Activity
3,3'-Diiodo-L-thyronine (T2) is a thyroid hormone derivative that has garnered attention for its potential biological activities and metabolic effects. This article explores the biological activity of T2, focusing on its mechanisms, effects on metabolism, and relevant research findings.
Overview of 3,3'-Diiodo-L-thyronine (T2)
T2 is a product of thyroid hormone metabolism, specifically derived from the deiodination of thyroxine (T4) and triiodothyronine (T3). It is considered to exert weaker thyromimetic effects compared to T3 but has been shown to influence various physiological processes, including energy metabolism and growth hormone production.
Binding Affinity and Receptor Interaction
Research indicates that T2 binds to thyroid hormone receptors (TRs), albeit with lower affinity than T3. In studies involving cultured rat pituitary cells, T2 demonstrated the ability to stimulate growth hormone production and glucose consumption, similar to T3 but requiring higher concentrations for equivalent effects .
Metabolic Effects
T2 has been implicated in several metabolic processes:
- Energy Metabolism : T2 administration resulted in increased metabolic rates and body temperature in animal models. It also influenced body composition by decreasing fat mass and increasing lean mass .
- Glucose Regulation : Studies have shown that T2 can stimulate glucose uptake in various cell types, suggesting a role in glucose homeostasis .
Case Studies and Experimental Data
- Growth Hormone Stimulation : In a study examining the effects of T2 on rat pituitary cells, it was found that T2 could stimulate growth hormone production at concentrations significantly higher than those required for T3. This suggests that while T2 may act as a weak agonist at TRs, it still plays a role in hormonal regulation .
- Metabolic Profiling : A feasibility study quantified the levels of T2 in human serum using mass spectrometry. The average concentration of 3,3'-T2 was found to be approximately 133 ± 15 pg/ml, indicating its presence in the circulation and potential physiological relevance .
Comparative Analysis of Thyroid Hormone Derivatives
Compound | Binding Affinity | Metabolic Effects | Concentration in Serum (pg/ml) |
---|---|---|---|
T3 | High | Strong stimulation of metabolism | Varies widely |
3,5-Diiodo-L-thyronine (3,5-T2) | Moderate | Modulates energy metabolism | 41 ± 5 |
3,3'-Diiodo-L-thyronine (T2) | Low | Weak agonist for TRs | 133 ± 15 |
Properties
IUPAC Name |
(2S)-2-amino-3-[4-(4-hydroxy-3-iodo(1,2,3,4,5,6-13C6)cyclohexa-1,3,5-trien-1-yl)oxy-3-iodophenyl]propanoic acid | |
---|---|---|
Source | PubChem | |
URL | https://pubchem.ncbi.nlm.nih.gov | |
Description | Data deposited in or computed by PubChem | |
InChI |
InChI=1S/C15H13I2NO4/c16-10-7-9(2-3-13(10)19)22-14-4-1-8(5-11(14)17)6-12(18)15(20)21/h1-5,7,12,19H,6,18H2,(H,20,21)/t12-/m0/s1/i2+1,3+1,7+1,9+1,10+1,13+1 | |
Source | PubChem | |
URL | https://pubchem.ncbi.nlm.nih.gov | |
Description | Data deposited in or computed by PubChem | |
InChI Key |
CPCJBZABTUOGNM-USQDWSSZSA-N | |
Source | PubChem | |
URL | https://pubchem.ncbi.nlm.nih.gov | |
Description | Data deposited in or computed by PubChem | |
Canonical SMILES |
C1=CC(=C(C=C1CC(C(=O)O)N)I)OC2=CC(=C(C=C2)O)I | |
Source | PubChem | |
URL | https://pubchem.ncbi.nlm.nih.gov | |
Description | Data deposited in or computed by PubChem | |
Isomeric SMILES |
C1=CC(=C(C=C1C[C@@H](C(=O)O)N)I)O[13C]2=[13CH][13C](=[13C]([13CH]=[13CH]2)O)I | |
Source | PubChem | |
URL | https://pubchem.ncbi.nlm.nih.gov | |
Description | Data deposited in or computed by PubChem | |
Molecular Formula |
C15H13I2NO4 | |
Source | PubChem | |
URL | https://pubchem.ncbi.nlm.nih.gov | |
Description | Data deposited in or computed by PubChem | |
DSSTOX Substance ID |
DTXSID60746356 | |
Record name | O-[4-Hydroxy-3-iodo(~13~C_6_)phenyl]-3-iodo-L-tyrosine | |
Source | EPA DSSTox | |
URL | https://comptox.epa.gov/dashboard/DTXSID60746356 | |
Description | DSSTox provides a high quality public chemistry resource for supporting improved predictive toxicology. | |
Molecular Weight |
531.03 g/mol | |
Source | PubChem | |
URL | https://pubchem.ncbi.nlm.nih.gov | |
Description | Data deposited in or computed by PubChem | |
CAS No. |
1217459-13-6 | |
Record name | O-[4-Hydroxy-3-iodo(~13~C_6_)phenyl]-3-iodo-L-tyrosine | |
Source | EPA DSSTox | |
URL | https://comptox.epa.gov/dashboard/DTXSID60746356 | |
Description | DSSTox provides a high quality public chemistry resource for supporting improved predictive toxicology. | |
Record name | 1217459-13-6 | |
Source | European Chemicals Agency (ECHA) | |
URL | https://echa.europa.eu/information-on-chemicals | |
Description | The European Chemicals Agency (ECHA) is an agency of the European Union which is the driving force among regulatory authorities in implementing the EU's groundbreaking chemicals legislation for the benefit of human health and the environment as well as for innovation and competitiveness. | |
Explanation | Use of the information, documents and data from the ECHA website is subject to the terms and conditions of this Legal Notice, and subject to other binding limitations provided for under applicable law, the information, documents and data made available on the ECHA website may be reproduced, distributed and/or used, totally or in part, for non-commercial purposes provided that ECHA is acknowledged as the source: "Source: European Chemicals Agency, http://echa.europa.eu/". Such acknowledgement must be included in each copy of the material. ECHA permits and encourages organisations and individuals to create links to the ECHA website under the following cumulative conditions: Links can only be made to webpages that provide a link to the Legal Notice page. | |
Disclaimer and Information on In-Vitro Research Products
Please be aware that all articles and product information presented on BenchChem are intended solely for informational purposes. The products available for purchase on BenchChem are specifically designed for in-vitro studies, which are conducted outside of living organisms. In-vitro studies, derived from the Latin term "in glass," involve experiments performed in controlled laboratory settings using cells or tissues. It is important to note that these products are not categorized as medicines or drugs, and they have not received approval from the FDA for the prevention, treatment, or cure of any medical condition, ailment, or disease. We must emphasize that any form of bodily introduction of these products into humans or animals is strictly prohibited by law. It is essential to adhere to these guidelines to ensure compliance with legal and ethical standards in research and experimentation.