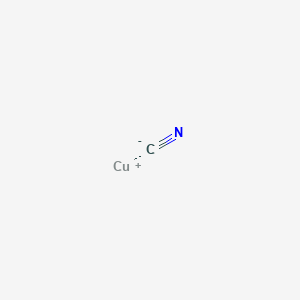
Copper(I) cyanide
Overview
Description
Copper(I) cyanide (CuCN) is an inorganic compound with the molecular formula CuCN and a molecular weight of 89.563 g/mol . It exists as a white to cream-colored powder with a melting point of 474 °C and a density of 2.92 g/mL at 25 °C . Structurally, CuCN forms polymeric chains in the solid state, where copper(I) centers are bridged by cyanide ligands in a linear coordination geometry . In aqueous solutions, Cu(I) predominantly forms tetra-coordinate complexes such as [Cu(CN)₂]⁻, [Cu(CN)₃]²⁻, and [Cu(CN)₄]³⁻, depending on cyanide concentration and pH .
CuCN is notable for its role in gold extraction processes, where it stabilizes cyanide ions in metallurgical solutions . It also serves as a precursor in organic synthesis, particularly in click chemistry and template reactions, such as the accidental synthesis of copper(II) phthalocyanine .
Preparation Methods
Copper(I) cyanide can be synthesized through several methods:
Synthetic Routes and Reaction Conditions: One common method involves the reaction of copper(II) sulfate with sodium cyanide, which produces this compound and sodium sulfate as by-products. The reaction is typically carried out in an aqueous solution[ \text{CuSO}_4 + 2\text{NaCN} \rightarrow \text{CuCN} + \text{Na}_2\text{SO}_4 ]
Industrial Production Methods: Industrially, this compound is produced by the reduction of copper(II) cyanide in the presence of a reducing agent such as sodium bisulfite.
Chemical Reactions Analysis
Copper(I) cyanide undergoes various types of chemical reactions:
Oxidation: It can be oxidized to copper(II) cyanide in the presence of oxidizing agents.
Reduction: this compound can be reduced to metallic copper using strong reducing agents.
Substitution: It participates in substitution reactions, such as the Rosenmund-von Braun reaction, where it reacts with aryl halides to form aryl nitriles.
Common Reagents and Conditions: Common reagents include sodium cyanide, potassium cyanide, and various oxidizing and reducing agents. The conditions often involve aqueous or polar organic solvents and controlled temperatures.
Major Products: Major products from these reactions include copper(II) cyanide, metallic copper, and various organic nitriles.
Scientific Research Applications
Copper(I) cyanide has a wide range of applications in scientific research:
Chemistry: It is used as a catalyst in organic synthesis, particularly in the formation of carbon-nitrogen bonds.
Biology: While not commonly used directly in biological research, its derivatives and complexes are studied for their potential biological activities.
Medicine: this compound itself is not widely used in medicine, but its complexes are explored for their antimicrobial and anticancer properties.
Industry: It is extensively used in electroplating processes for copper, iron, silver, brass, and copper-tin alloys.
Mechanism of Action
The mechanism by which copper(I) cyanide exerts its effects involves its ability to form complexes with various ligands. In catalytic processes, it often acts by facilitating the transfer of cyanide ions to organic substrates, thereby forming nitriles. The molecular targets and pathways involved include the formation of intermediate complexes with transition metals, which then undergo further transformations to yield the desired products .
Comparison with Similar Compounds
Structural Features and Coordination Chemistry
Table 1: Structural Comparison of Metal Cyanides
CuCN’s linear polymeric structure contrasts with the 3D frameworks of Cd(CN)₂ and the octahedral coordination in hexacyanoferrates. The shorter Cu–C bonds (1.82–1.87 Å) compared to Ag–C (2.05 Å) reflect the smaller ionic radius of Cu(I) .
Stability and Reactivity
Table 2: Stability Constants and Decomposition Pathways
CuCN complexes exhibit moderate stability in solution but are less stable than Fe(III) cyanides. Under alkaline conditions, CuCN forms stable [Cu(CN)₄]³⁻, while acidic conditions promote decomposition to HCN and Cu²⁺ .
Photoluminescence Properties
CuCN’s luminescence arises from electronic transitions in its 1-D chains. For example, potassium-capped [Cu₃(CN)₄]⁻ chains emit at 330 nm due to structural distortions in the excited state . In contrast, Cd(CN)₂ lacks luminescence due to its rigid 3D framework, while AgCN shows weaker emission because of less pronounced metal-ligand bonding interactions .
Table 3: Hazard Comparison of Cyanides
CuCN is highly toxic, with hazards comparable to KCN but less volatile than HCN. Proper handling requires PPE and ventilation to avoid inhalation or dermal exposure .
Biological Activity
Copper(I) cyanide (CuCN) is a compound that exhibits significant biological activity, particularly in the context of toxicity and environmental impact. This article delves into its biological properties, mechanisms of action, and effects on various organisms, including case studies and data tables to illustrate key findings.
This compound is a coordination compound formed from copper and cyanide ions. It has been utilized in various industrial applications, including electroplating and as a biocide in marine paints. Its chemical structure can be represented as CCuN, indicating the presence of copper in the +1 oxidation state combined with cyanide .
Toxicity Profile:
- LD50 Values: The oral LD50 for rats is approximately 1265 mg/kg, indicating moderate toxicity .
- Acute Toxicity: In aquatic environments, CuCN has been shown to have an LC50 of 0.76 mg/L for the freshwater fish Catla catla, leading to erratic swimming patterns and increased gill flapping among affected individuals .
The biological activity of this compound is largely attributed to its ability to release cyanide ions upon decomposition. Cyanide acts as a potent inhibitor of cytochrome c oxidase in the mitochondrial electron transport chain, which disrupts ATP production, particularly affecting tissues reliant on aerobic respiration such as the brain and heart .
Key Mechanisms:
- Cyanide Binding: Cyanide binds to ferric iron in cytochrome c oxidase, preventing electron transfer to oxygen and halting cellular respiration.
- Oxidative Stress: Copper ions can induce oxidative stress through the generation of reactive oxygen species (ROS), which may lead to cellular apoptosis via pathways involving sphingomyelinase activation and ceramide production .
Biological Effects on Organisms
This compound's effects extend beyond direct toxicity; it also influences metabolic processes in various organisms.
Case Studies:
-
Fish Toxicity Study:
- A study on Catla catla revealed that exposure to CuCN resulted in behavioral changes such as hyperactivity and loss of equilibrium. The study quantitatively assessed these changes over 96 hours, demonstrating significant alterations in swimming behavior correlated with copper cyanide concentration .
Time (h) Erratic Swimming Hyperactivity Loss of Balance 24 + + - 48 ++ ++ + 72 +++ +++ ++ 96 ++++ ++++ ++++ - Impact on Metabolic Enzymes:
Environmental Implications
The presence of this compound in aquatic environments poses significant risks to biodiversity. Its toxicity not only affects individual species but can also disrupt entire ecosystems by altering food webs and nutrient cycling.
Biodegradation Studies
Recent studies have explored the potential for microbial degradation of cyanides complexed with metals like copper. A bacterial consortium isolated from contaminated sites demonstrated varying efficacy in degrading free and complexed cyanides, highlighting the role of environmental factors in biodegradation processes .
Q & A
Q. Basic: What are the optimal conditions for synthesizing Copper(I) cyanide in a laboratory setting, and how can purity be validated?
Methodological Answer:
this compound (CuCN) is typically synthesized via the reaction of copper(I) salts with cyanide ions under controlled pH and temperature. A common method involves adding potassium cyanide to a copper sulfate solution in an inert atmosphere to prevent oxidation to Cu(II). Key parameters include:
- pH control (acidic conditions stabilize CuCN but require careful cyanide handling to avoid HCN gas release) .
- Temperature regulation (ambient to 60°C, depending on reaction kinetics).
- Purification : Recrystallization from ammonia solutions or ethanol washes to remove impurities.
- Purity validation : Use X-ray diffraction (XRD) for crystallographic confirmation, elemental analysis (C, N, Cu quantification), and FTIR to verify absence of cyanide degradation products .
Q. Advanced: How can inconsistencies in reported crystallographic data for this compound be resolved through advanced analytical techniques?
Methodological Answer:
Discrepancies in crystallographic data often arise from polymorphic variations or incomplete structural characterization. To resolve these:
- High-resolution XRD or neutron diffraction can distinguish between cubic and hexagonal polymorphs, clarifying bonding geometries .
- Computational modeling (DFT calculations) predicts stable configurations, cross-referenced with experimental data .
- In situ Raman spectroscopy monitors phase transitions under varying temperatures/pressures.
- Meta-analysis of existing datasets using standardized refinement protocols (e.g., Rietveld method) minimizes instrumental bias .
Q. Basic: What safety protocols are critical when handling this compound to mitigate acute and chronic exposure risks?
Methodological Answer:
CuCN poses acute toxicity (H302, H315, H319) and chronic hazards (H372, H373, H410) . Essential protocols include:
- Engineering controls : Local exhaust ventilation and sealed reaction systems to minimize dust/aerosol formation .
- Personal Protective Equipment (PPE) : Nitrile gloves (JIS T 8116), chemical goggles (JIS T 8147), and lab coats.
- Emergency response : Immediate eye rinsing (15+ minutes with saline) and medical consultation for ingestion/inhalation .
- Waste management : Neutralize cyanide-containing waste with hypochlorite before disposal to prevent aquatic toxicity .
Q. Advanced: What methodologies are effective in assessing the environmental persistence and ecotoxicological impact of this compound in aquatic systems?
Methodological Answer:
- Persistence studies : Conduct OECD 309 tests to measure hydrolysis and photodegradation rates in water under varying pH and light conditions .
- Bioaccumulation assays : Expose model organisms (e.g., Daphnia magna) to CuCN-spiked water, quantifying Cu uptake via ICP-MS and assessing chronic toxicity (mortality, reproduction rates) .
- Sediment interaction analysis : Use sequential extraction to evaluate CuCN mobility in soil-water systems, correlating with organic matter content and redox potential .
- Computational ecotoxicology : QSAR models predict toxicity thresholds for unstudied species, validated with experimental LC50 data .
Q. Data Analysis: How should researchers address discrepancies in thermodynamic stability data of this compound across different studies?
Methodological Answer:
- Systematic uncertainty analysis : Identify measurement variables (e.g., calorimetric vs. electrochemical ΔG determinations) and propagate errors using Monte Carlo simulations .
- Cross-validation : Compare DSC (differential scanning calorimetry) results with solubility studies in ammonia/water systems to reconcile stability contradictions .
- Data normalization : Adjust for differences in experimental conditions (e.g., ionic strength, temperature) using activity coefficient models (Pitzer equations) .
Q. Experimental Design: What strategies ensure reproducibility in this compound-based catalytic studies, particularly regarding precursor interactions?
Methodological Answer:
- Precursor characterization : Pre-treat copper precursors (e.g., CuCl) with reducing agents (ascorbic acid) to eliminate Cu(II) impurities that alter reaction pathways .
- Inert atmosphere standardization : Use Schlenk lines or gloveboxes (O₂ < 1 ppm) to prevent CuCN oxidation during catalysis .
- Kinetic profiling : Monitor reaction progress via in situ UV-Vis or NMR to identify intermediate species influencing reproducibility .
- Collaborative validation : Share protocols via open-access platforms (e.g., protocols.io ) for multi-lab verification, addressing batch-to-batch variability .
Q. Advanced: How can mechanistic studies differentiate between ligand-bridged vs. direct Cu-CN bonding in this compound complexes?
Methodological Answer:
- EXAFS spectroscopy : Analyze Cu K-edge spectra to determine coordination numbers and bond distances, distinguishing bridging vs. terminal cyanide ligands .
- Magnetic susceptibility measurements : Paramagnetic Cu(II) impurities indicate oxidation, while diamagnetic CuCN confirms Cu(I)-CN bonding .
- Theoretical simulations : CASSCF calculations model electronic structures, identifying d-orbital contributions to bonding .
Properties
CAS No. |
544-92-3 |
---|---|
Molecular Formula |
CCuN |
Molecular Weight |
89.56 g/mol |
IUPAC Name |
copper(1+);cyanide |
InChI |
InChI=1S/CN.Cu/c1-2;/q-1;+1 |
InChI Key |
DOBRDRYODQBAMW-UHFFFAOYSA-N |
SMILES |
[C-]#N.[Cu+] |
Canonical SMILES |
[C-]#N.[Cu+] |
boiling_point |
Decomposes |
Color/Form |
White monoclinic prisms or green orthorhombic crystals White to cream-colored powder, colorless or dark green orthorhombic crystals or dark red monoclinic crystals Pale cream powde |
density |
2.92 at 68 °F (USCG, 1999) 2.9 |
melting_point |
883 °F (NTP, 1992) 474 °C |
Key on ui other cas no. |
544-92-3 |
physical_description |
Copper cyanide appears as a green powder. Insoluble in water. Toxic by skin absorption, through open wounds, by ingestion, and by inhalation of hydrogen cyanide that arises from slight decomposition. Produces toxic oxides of nitrogen in fires. DryPowder; OtherSolid, Liquid |
Pictograms |
Acute Toxic; Environmental Hazard |
solubility |
Practically insol in water, alcohol, cold dil acids; sol in ammonium hydroxide, sol in alkali cyanide solutions because of formation of stable cyanocuprate(I) ions Soluble in potassium cyanide solution; insoluble in water and ethanol Soluble in sodium ... cyanide, hydrochloric acid ... . |
Synonyms |
Copper Cyanide; Copper Monocyanide; Copper(1+) Cyanide; Copper(I) Cyanide; Cupricin; Cuprous Cyanide |
Origin of Product |
United States |
Retrosynthesis Analysis
AI-Powered Synthesis Planning: Our tool employs the Template_relevance Pistachio, Template_relevance Bkms_metabolic, Template_relevance Pistachio_ringbreaker, Template_relevance Reaxys, Template_relevance Reaxys_biocatalysis model, leveraging a vast database of chemical reactions to predict feasible synthetic routes.
One-Step Synthesis Focus: Specifically designed for one-step synthesis, it provides concise and direct routes for your target compounds, streamlining the synthesis process.
Accurate Predictions: Utilizing the extensive PISTACHIO, BKMS_METABOLIC, PISTACHIO_RINGBREAKER, REAXYS, REAXYS_BIOCATALYSIS database, our tool offers high-accuracy predictions, reflecting the latest in chemical research and data.
Strategy Settings
Precursor scoring | Relevance Heuristic |
---|---|
Min. plausibility | 0.01 |
Model | Template_relevance |
Template Set | Pistachio/Bkms_metabolic/Pistachio_ringbreaker/Reaxys/Reaxys_biocatalysis |
Top-N result to add to graph | 6 |
Feasible Synthetic Routes
Disclaimer and Information on In-Vitro Research Products
Please be aware that all articles and product information presented on BenchChem are intended solely for informational purposes. The products available for purchase on BenchChem are specifically designed for in-vitro studies, which are conducted outside of living organisms. In-vitro studies, derived from the Latin term "in glass," involve experiments performed in controlled laboratory settings using cells or tissues. It is important to note that these products are not categorized as medicines or drugs, and they have not received approval from the FDA for the prevention, treatment, or cure of any medical condition, ailment, or disease. We must emphasize that any form of bodily introduction of these products into humans or animals is strictly prohibited by law. It is essential to adhere to these guidelines to ensure compliance with legal and ethical standards in research and experimentation.