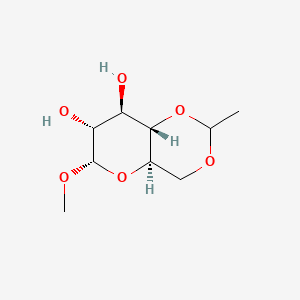
Methyl-4,6-O-ethylidene-alpha-D-glucopyranoside
- Click on QUICK INQUIRY to receive a quote from our team of experts.
- With the quality product at a COMPETITIVE price, you can focus more on your research.
Overview
Description
“Methyl-4,6-O-ethylidene-alpha-D-glucopyranoside” is a heterocyclic organic compound with the molecular formula C9H16O6 and a molecular weight of 220.21974 . It is used for research purposes .
Synthesis Analysis
A saccharide-based ligand suitable for metal binding has been synthesized using 4,6-O-ethylidene-α-D-glucopyranose (4,6-O-EGP) and anthranilic acid . Analogous glycosylamines with chloro and fluoro ortho substitutions have also been synthesized and characterized .Molecular Structure Analysis
The molecular structure of “Methyl-4,6-O-ethylidene-alpha-D-glucopyranoside” consists of nine carbon atoms, sixteen hydrogen atoms, and six oxygen atoms .Chemical Reactions Analysis
“Methyl-4,6-O-ethylidene-alpha-D-glucopyranoside” is used as a chiral building block and an important intermediate in the preparation of different sugars . It is also used as an intermediate in chemical and pharmaceutical research .Physical And Chemical Properties Analysis
“Methyl-4,6-O-ethylidene-alpha-D-glucopyranoside” is a white powder or fused solid . It is soluble in water, acetone, benzene, and ethyl ether . The recommended storage temperature is 2 - 8°C .Mechanism of Action
Safety and Hazards
Future Directions
properties
IUPAC Name |
(4aR,6S,7R,8R,8aS)-6-methoxy-2-methyl-4,4a,6,7,8,8a-hexahydropyrano[3,2-d][1,3]dioxine-7,8-diol |
Source
|
---|---|---|
Source | PubChem | |
URL | https://pubchem.ncbi.nlm.nih.gov | |
Description | Data deposited in or computed by PubChem | |
InChI |
InChI=1S/C9H16O6/c1-4-13-3-5-8(14-4)6(10)7(11)9(12-2)15-5/h4-11H,3H2,1-2H3/t4?,5-,6-,7-,8-,9+/m1/s1 |
Source
|
Source | PubChem | |
URL | https://pubchem.ncbi.nlm.nih.gov | |
Description | Data deposited in or computed by PubChem | |
InChI Key |
BSBOLIVKPWXZAN-KTTWVITLSA-N |
Source
|
Source | PubChem | |
URL | https://pubchem.ncbi.nlm.nih.gov | |
Description | Data deposited in or computed by PubChem | |
Canonical SMILES |
CC1OCC2C(O1)C(C(C(O2)OC)O)O |
Source
|
Source | PubChem | |
URL | https://pubchem.ncbi.nlm.nih.gov | |
Description | Data deposited in or computed by PubChem | |
Isomeric SMILES |
CC1OC[C@@H]2[C@@H](O1)[C@@H]([C@H]([C@H](O2)OC)O)O |
Source
|
Source | PubChem | |
URL | https://pubchem.ncbi.nlm.nih.gov | |
Description | Data deposited in or computed by PubChem | |
Molecular Formula |
C9H16O6 |
Source
|
Source | PubChem | |
URL | https://pubchem.ncbi.nlm.nih.gov | |
Description | Data deposited in or computed by PubChem | |
Molecular Weight |
220.22 g/mol |
Source
|
Source | PubChem | |
URL | https://pubchem.ncbi.nlm.nih.gov | |
Description | Data deposited in or computed by PubChem | |
Product Name |
Methyl-4,6-O-ethylidene-alpha-D-glucopyranoside |
Disclaimer and Information on In-Vitro Research Products
Please be aware that all articles and product information presented on BenchChem are intended solely for informational purposes. The products available for purchase on BenchChem are specifically designed for in-vitro studies, which are conducted outside of living organisms. In-vitro studies, derived from the Latin term "in glass," involve experiments performed in controlled laboratory settings using cells or tissues. It is important to note that these products are not categorized as medicines or drugs, and they have not received approval from the FDA for the prevention, treatment, or cure of any medical condition, ailment, or disease. We must emphasize that any form of bodily introduction of these products into humans or animals is strictly prohibited by law. It is essential to adhere to these guidelines to ensure compliance with legal and ethical standards in research and experimentation.