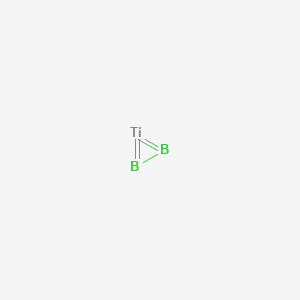
Titanium boride
- Click on QUICK INQUIRY to receive a quote from our team of experts.
- With the quality product at a COMPETITIVE price, you can focus more on your research.
Overview
Description
Titanium boride is a ceramic material known for its exceptional hardness, thermal stability, and electrical conductivity. It is a member of the boride family, which consists of compounds formed between boron and metals. This compound is particularly notable for its high melting point and resistance to chemical attack, making it a valuable material in various industrial applications.
Synthetic Routes and Reaction Conditions:
Carbothermal Reduction Method: This method involves the reduction of titanium dioxide and boron oxide with carbon at high temperatures. The reaction typically occurs in a vacuum or inert atmosphere to prevent oxidation.
Self-Propagating High-Temperature Synthesis (SHS): This method utilizes the exothermic reaction between titanium and boron powders to produce this compound. The reaction is initiated by an external heat source and propagates through the reactant mixture.
Mechanochemical Reaction Method: This involves the mechanical activation of titanium and boron powders through high-energy ball milling, which induces a reaction between the components.
Vapor Deposition Method: this compound can be deposited as a thin film using chemical vapor deposition (CVD) or physical vapor deposition (PVD) techniques.
Industrial Production Methods:
Hot Pressing (HP): This method involves pressing titanium and boron powders at high temperatures and pressures to form dense this compound components.
Spark Plasma Sintering (SPS): This technique uses pulsed electric currents to sinter titanium and boron powders, resulting in high-density this compound materials.
Types of Reactions:
Oxidation: this compound can undergo oxidation at high temperatures, forming titanium dioxide and boron oxide.
Reduction: this compound can be reduced to titanium and boron using strong reducing agents such as magnesium or calcium.
Substitution: this compound can participate in substitution reactions where boron atoms are replaced by other elements, altering its properties.
Common Reagents and Conditions:
Oxidation: Typically occurs at temperatures above 800°C in the presence of oxygen.
Reduction: Requires strong reducing agents and high temperatures, often conducted in a vacuum or inert atmosphere.
Substitution: Involves the use of reactive elements or compounds that can replace boron atoms in the this compound lattice.
Major Products:
Oxidation: Titanium dioxide and boron oxide.
Reduction: Titanium and elemental boron.
Substitution: Modified this compound compounds with altered properties.
Scientific Research Applications
Titanium boride has a wide range of applications in scientific research due to its unique properties:
Chemistry: Used as a catalyst in various chemical reactions, including hydrogen evolution and oxygen reduction reactions.
Biology: Investigated for its potential use in biomedical implants due to its biocompatibility and mechanical strength.
Medicine: Explored for use in drug delivery systems and as a component in medical devices.
Industry: Utilized in the production of cutting tools, wear-resistant coatings, and armor materials. It is also used in the manufacture of crucibles and other high-temperature components.
Mechanism of Action
The mechanism by which titanium boride exerts its effects is primarily related to its structural and electronic properties:
Molecular Targets: this compound interacts with various molecular species through its surface, which can be modified to enhance catalytic activity.
Pathways Involved: The material’s high hardness and thermal stability make it effective in applications requiring resistance to wear and high temperatures. Its electrical conductivity allows it to participate in electrochemical reactions, making it useful in energy storage and conversion applications.
Comparison with Similar Compounds
Titanium boride is often compared with other borides and carbides due to its similar properties:
Similar Compounds: Titanium carbide, zirconium boride, hafnium boride, and tungsten carbide.
Uniqueness: this compound stands out due to its combination of high hardness, thermal stability, and electrical conductivity. Unlike some other borides, it is relatively easy to produce and process, making it more accessible for industrial applications.
Chemical Reactions Analysis
Reactions with Acids and Bases
- Acids : Titanium diboride is more reactive to strongly acidic solutions than its corresponding carbides and nitrides . TiB2 can displace hydrogen ions (H+) from strong acids, forming titanous ions (Ti+3) .
- Bases : While generally stable, titanium boride can react with fused potassium hydroxide (KOH) at high temperatures .
High-Temperature Reactions
At high temperatures, this compound can undergo several reactions:
- Oxidation : While TiB2 is relatively resistant to oxidation, it will oxidize at elevated temperatures .
- Synthesis : TiB2 is typically synthesized through high-temperature reactions, such as direct sintering using elemental powders . Self-propagating high-temperature synthesis (SHS) can also produce titanium borides from titanium and boron mixtures under dynamic vacuum conditions .
Reactions with Other Materials
- Metal Salt Solutions : Titanium diboride can reduce metal salt solutions, such as copper sulfate (CuSO4), to their corresponding free metals . When exposed to acidified copper sulfate solution, TiB2 results in the formation of an adherent copper deposit .
- Nitrogen : In reactions involving nitrogen, approximately 60% of the total nitrogen forms ammonium ions (NH4+), with the remaining 40% evolving as nitrogen gas (N2) .
Potential Hazards
This compound may emit toxic fumes when heated to decomposition . On contact with moisture, water, or acids, it may emit spontaneously flammable hydrides .
Experimental Observations
Q & A
Basic Research Questions
Q. What experimental methods are most effective for synthesizing high-purity titanium boride (TiB2), and how do processing parameters influence phase purity?
- Methodological Answer : TiB2 synthesis via solid-state reaction (e.g., boron and titanium precursors) requires precise stoichiometric control and inert atmospheres to prevent oxidation. Characterization using XRD and SEM-EDS is critical to confirm phase purity . For example, deviations in sintering temperatures above 1500°C may induce unintended silicide phases in composites, complicating phase identification .
Q. How can researchers validate the mechanical properties of TiB2-based composites, and what standardized tests are recommended?
- Methodological Answer : Nanoindentation and Vickers hardness tests are standard for assessing hardness, while three-point bending tests evaluate flexural strength. Tribological studies (e.g., pin-on-disk) quantify wear resistance. Note that discrepancies in hardness values (~25–35 GPa) may arise from porosity or secondary phases, necessitating microstructural analysis via TEM .
Q. What spectroscopic techniques are optimal for analyzing TiB2’s thermal stability under extreme conditions?
- Methodological Answer : High-temperature XRD and thermogravimetric analysis (TGA) under controlled atmospheres (e.g., argon) reveal phase transitions and oxidation thresholds. For instance, TiB2 exhibits stability up to 1100°C in inert environments but degrades rapidly in oxygen-rich settings .
Advanced Research Questions
Q. How can computational modeling address contradictions in experimental data on TiB2’s fracture toughness?
- Methodological Answer : Molecular dynamics (MD) simulations and finite element analysis (FEA) can resolve discrepancies by modeling crack propagation at grain boundaries. For example, MD studies show that TiB2’s brittleness under tensile stress correlates with boron vacancy density, a factor often overlooked in empirical studies .
Q. What strategies mitigate interfacial incompatibility in TiB2-ceramic composites, and how should these be experimentally validated?
- Methodological Answer : Introducing transition layers (e.g., SiC or Al2O3) via chemical vapor deposition (CVD) improves adhesion. Cross-sectional SEM and EDS mapping of interfaces post-sintering can validate bonding efficacy. However, residual stresses from coefficient of thermal expansion (CTE) mismatches require synchrotron-based strain analysis .
Q. How does the incorporation of machine learning enhance the design of TiB2-based composites for aerospace applications?
- Methodological Answer : Neural networks trained on datasets of mechanical properties (e.g., hardness, fatigue resistance) can predict optimal ceramic reinforcement ratios. For instance, ML models at the University of Iowa identified TiB2-SiC composites with 15–20 vol% SiC as ideal for balancing wear resistance and machinability .
Q. What advanced microscopy techniques resolve ambiguities in TiB2’s grain boundary diffusion mechanisms during sintering?
- Methodological Answer : Atom probe tomography (APT) and electron backscatter diffraction (EBSD) provide nanoscale insights into boron diffusion pathways. A 2023 study using APT revealed that carbon impurities segregate at grain boundaries, impeding densification—a critical consideration for spark plasma sintering (SPS) protocols .
Q. Data Contradiction Analysis
Q. Why do studies report conflicting values for TiB2’s thermal conductivity, and how can methodologies be standardized?
- Methodological Answer : Variations arise from differences in sample porosity and measurement techniques (e.g., laser flash vs. steady-state methods). A 2024 review recommends using laser flash analysis with fully dense samples (>98% theoretical density) and reporting anisotropy ratios for single-crystal vs. polycrystalline TiB2 .
Q. How should researchers interpret divergent results in TiB2’s corrosion resistance across acidic and alkaline environments?
- Methodological Answer : Electrochemical impedance spectroscopy (EIS) and potentiodynamic polarization in pH-controlled solutions are essential. Contradictions often stem from surface passivation effects; for example, alkaline media promote TiO2 passivation layers, whereas acidic conditions dissolve these layers, accelerating corrosion .
Q. Experimental Design Guidelines
Q. What controls are critical when designing in vitro studies on TiB2’s biocompatibility for biomedical implants?
- Methodological Answer : Use ISO 10993-5 standards for cytotoxicity assays (e.g., fibroblast viability tests). Surface roughness (Ra < 0.5 µm) and sterilization methods (autoclave vs. gamma irradiation) must be standardized, as these factors significantly influence inflammatory responses .
Q. How can researchers optimize DOE (Design of Experiments) for high-throughput screening of TiB2 composite formulations?
- Methodological Answer : Taguchi methods with L18 orthogonal arrays efficiently test variables like sintering temperature (1400–1600°C), pressure (20–50 MPa), and reinforcement ratios. Response surface methodology (RSM) then models interactions between parameters to maximize hardness and minimize porosity .
Properties
CAS No. |
12045-63-5 |
---|---|
Molecular Formula |
B2Ti |
Molecular Weight |
69.49 g/mol |
IUPAC Name |
1,2-dibora-3λ4-titanacyclopropa-2,3-diene |
InChI |
InChI=1S/2B.Ti |
InChI Key |
QYEXBYZXHDUPRC-UHFFFAOYSA-N |
SMILES |
B1=[Ti]=B1 |
Canonical SMILES |
[B].[B].[Ti] |
Origin of Product |
United States |
Disclaimer and Information on In-Vitro Research Products
Please be aware that all articles and product information presented on BenchChem are intended solely for informational purposes. The products available for purchase on BenchChem are specifically designed for in-vitro studies, which are conducted outside of living organisms. In-vitro studies, derived from the Latin term "in glass," involve experiments performed in controlled laboratory settings using cells or tissues. It is important to note that these products are not categorized as medicines or drugs, and they have not received approval from the FDA for the prevention, treatment, or cure of any medical condition, ailment, or disease. We must emphasize that any form of bodily introduction of these products into humans or animals is strictly prohibited by law. It is essential to adhere to these guidelines to ensure compliance with legal and ethical standards in research and experimentation.