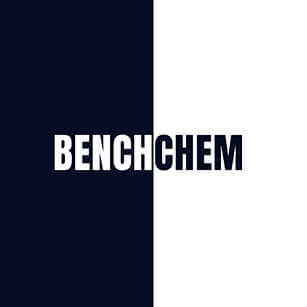
TERBIUM BROMIDE
- Click on QUICK INQUIRY to receive a quote from our team of experts.
- With the quality product at a COMPETITIVE price, you can focus more on your research.
Overview
Description
Terbium bromide, also known as terbium(III) bromide, is a crystalline chemical compound with the formula TbBr₃. It is a white powder that is soluble in water and has a molar mass of 398.637 g/mol. This compound is part of the lanthanide series and is used in various scientific and industrial applications due to its unique properties .
Preparation Methods
Synthetic Routes and Reaction Conditions: Terbium bromide can be synthesized by heating terbium metal or terbium(III) oxide with ammonium bromide. The reaction is as follows:
Tb2O3+6NH4Br→2TbBr3+6NH3+3H2O
A solution of terbium(III) bromide can crystallize as a hexahydrate. When heating the hexahydrate, it will dehydrate and produce some terbium oxybromide (TbOBr) .
Industrial Production Methods: In industrial settings, this compound is often produced by reacting terbium metal with bromine gas. The reaction is carried out in a controlled environment to ensure the purity of the product .
Types of Reactions:
Oxidation: this compound can undergo oxidation reactions, forming terbium oxide (Tb₄O₇) when exposed to air.
Reduction: It can be reduced back to terbium metal under specific conditions.
Common Reagents and Conditions:
Oxidation: Exposure to air or oxygen.
Reduction: High-temperature reduction using hydrogen gas.
Substitution: Reaction with halogen gases like chlorine or iodine.
Major Products Formed:
Oxidation: Terbium oxide (Tb₄O₇)
Reduction: Terbium metal (Tb)
Substitution: Terbium chloride (TbCl₃), Terbium iodide (TbI₃).
Scientific Research Applications
Luminescent Materials
Terbium Bromide in Luminescent Applications
Terbium ions are known for their luminescent properties, which make them valuable in the development of phosphors and luminescent materials. This compound can be incorporated into various matrices to enhance their optical properties.
- Hydroxyapatite Doping : Research has demonstrated that doping hydroxyapatite with terbium ions significantly improves its luminescence. The incorporation of terbium ions into the hydroxyapatite structure results in materials that are biocompatible and exhibit strong green fluorescence, suitable for biomedical imaging applications .
- Nanoparticle Synthesis : The synthesis of terbium-doped silica nanoparticles has shown potential for use in bioimaging due to their excellent luminescent properties. These nanoparticles can serve as contrast agents in magnetic resonance imaging (MRI) and other imaging modalities .
Biomedical Applications
Targeted Radionuclide Therapy
Terbium isotopes, particularly terbium-161 and terbium-149, have been investigated for use in targeted radionuclide therapy for cancer treatment.
- Folate Receptor Targeting : A study involving the use of terbium-149 labeled with a folate conjugate demonstrated significant anticancer efficacy against folate receptor-positive tumors. The results indicated a marked delay in tumor growth and improved survival rates in treated mice compared to controls .
- Prostate Cancer Therapy : Terbium-161 has been evaluated for its effectiveness in treating prostate cancer using prostate-specific membrane antigen (PSMA) targeting. Preclinical studies indicated that terbium-161 provided superior tumor response compared to lutetium-177, highlighting its potential as a more effective therapeutic option .
Environmental Applications
Heavy Metal Ion Removal
Research indicates that terbium compounds can be utilized in bioremediation processes to remove heavy metals from contaminated environments. This compound can facilitate the biosorption of toxic metals through its unique chemical properties.
- Biosorption Studies : Studies have shown that microorganisms can effectively utilize terbium ions to enhance the biosorption capacity for heavy metals such as lead and chromium. This application is crucial for developing sustainable methods for environmental cleanup .
Optical Devices
Applications in Optoelectronics
This compound's optical properties make it suitable for use in optoelectronic devices.
- Phosphor Materials : Terbium-doped phosphors are widely used in display technologies and lighting applications due to their efficient green emission. The incorporation of terbium into phosphor matrices enhances the brightness and color purity of displays .
Mechanism of Action
The mechanism of action of terbium bromide primarily involves its interaction with other molecules through its bromide ions. In biological systems, terbium ions can interact with cellular components, leading to bioadsorption and recovery of rare earth elements. The molecular targets and pathways involved include amino, hydroxyl, methyl, and phosphate groups on the surface of spores, which facilitate the adsorption process .
Comparison with Similar Compounds
- Terbium Chloride (TbCl₃)
- Terbium Iodide (TbI₃)
- Terbium Fluoride (TbF₃)
Comparison:
- Solubility: Terbium bromide is more soluble in water compared to terbium fluoride.
- Reactivity: this compound reacts similarly to terbium chloride and terbium iodide with halogens to form corresponding terbium halides .
- Applications: While terbium chloride and terbium iodide are also used in doping studies and fluorescence analysis, this compound is preferred for its higher solubility and ease of handling .
This compound stands out due to its unique combination of solubility, reactivity, and application potential, making it a valuable compound in various scientific and industrial fields.
Biological Activity
Terbium bromide (TbBr₃) is a compound of terbium, a rare earth element, and bromine. While it has applications in various fields such as electronics and materials science, its biological activity is a relatively underexplored area. This article synthesizes current research findings on the biological activity of this compound, focusing on its potential applications in medical and biological contexts.
Overview of this compound
Terbium is a member of the lanthanide series and exhibits unique electronic and photonic properties. Terbium compounds, including this compound, are known for their luminescent properties, making them valuable in various applications such as phosphors for lighting and displays. However, their biological implications are gaining attention due to their potential use in medical imaging and targeted therapies.
1. Fluorescent Properties and Applications
Terbium ions (Tb³⁺) are recognized for their fluorescence capabilities, which can be harnessed for biological imaging. Research indicates that Tb³⁺ ions can be complexed with organic ligands to enhance their luminescent properties. For instance, studies have shown that the complexation of Tb³⁺ with 1,10-phenanthroline and ascorbic acid results in significant fluorescence emission at specific wavelengths (489 nm, 544 nm) due to electronic transitions within the terbium ion . This property is particularly useful in biological assays where sensitive detection of biomolecules is required.
2. Cytotoxicity and Cell Viability
Recent studies have explored the cytotoxic effects of terbium compounds on various cell lines. For example, research involving terbium-161 (a radionuclide derived from terbium) demonstrated its effectiveness in reducing tumor cell viability compared to other isotopes like lutetium-177 . In vitro studies indicated that exposure to terbium-161 significantly decreased the survival rates of AR42J tumor cells, suggesting a potential application in cancer therapy .
In another study focusing on the effects of terbium ions on hydroxyapatite (HAp), it was found that doping HAp with Tb³⁺ ions enhanced its luminescent properties while maintaining cytocompatibility with MC3T3-E1 and A549 cells . This suggests that terbium-doped materials could be utilized in biomedical applications without adversely affecting cell viability.
Case Study 1: Radionuclide Therapy
A comparative study investigated the use of terbium-161 in PSMA-targeted radionuclide therapy for prostate cancer. The study found that mice treated with terbium-161 exhibited a median survival increase from 19 days (control) to 65 days at certain dosages, indicating its superior therapeutic efficacy over lutetium-177 . The dosimetry analysis revealed that terbium-161 had a higher energy deposition in tumors compared to its counterparts.
Treatment Type | Median Survival (Days) | Dosage (MBq/mouse) |
---|---|---|
Control | 19 | - |
Terbium-161 | 65 | 10 |
Lutetium-177 | 36 | 10 |
Case Study 2: Fluorescence in Biological Detection
In another application, researchers utilized terbium complexes for molecular detection. The study highlighted how the fluorescence efficiency of Tb(III) complexes could be influenced by surfactants, enhancing their stability and detection capabilities in biological samples . This property allows for the development of sensitive biosensors that can detect low concentrations of biomolecules.
Q & A
Basic Research Questions
Q. What are the optimal synthesis methods for terbium bromide in laboratory settings, and how do reaction conditions influence crystal purity?
- Methodology : Use solid-state reactions (e.g., Tb₂O₃ + NH₄Br → TbBr₃ + byproducts at 400–600°C under inert gas) or solution-based techniques (e.g., precipitation in HBr). Monitor purity via X-ray diffraction (XRD) and energy-dispersive X-ray spectroscopy (EDX). Key variables include temperature gradients, stoichiometric ratios, and reaction time .
- Data Validation : Compare lattice parameters (e.g., hexagonal vs. monoclinic phases) against the Inorganic Crystal Structure Database (ICSD) to resolve phase inconsistencies .
Q. How do vibrational spectroscopy (Raman/IR) and thermal analysis (TGA/DSC) characterize this compound’s stability and hydration states?
- Methodology : Assign vibrational modes (e.g., Tb–Br stretching at 200–300 cm⁻¹) and correlate with hydration levels. Use thermogravimetric analysis (TGA) to quantify water loss (e.g., TbBr₃·6H₂O → TbBr₃ at ~150°C) .
- Contradiction Analysis : Address discrepancies in reported decomposition temperatures by standardizing heating rates (e.g., 5°C/min under Argon) .
Advanced Research Questions
Q. What computational frameworks (DFT, MD) best predict this compound’s electronic structure and ligand-field effects in coordination complexes?
- Methodology : Apply density functional theory (DFT) with Hubbard-U corrections to model 4f-electron interactions. Compare simulated UV-Vis spectra (e.g., ⁷F₆ → ⁵D₄ transitions) with experimental data .
- Data Gaps : Resolve discrepancies in calculated vs. observed magnetic moments by refining spin-orbit coupling parameters .
Q. How can synchrotron X-ray absorption spectroscopy (XAS) resolve local coordination environments in amorphous this compound thin films?
- Experimental Design : Use EXAFS at the Tb L₃-edge to quantify bond distances (Tb–Br ≈ 2.8 Å) and disorder parameters. Cross-validate with pair distribution function (PDF) analysis .
- Challenges : Address beam-induced sample degradation by optimizing flux density and cryogenic cooling .
Q. What mechanisms explain discrepancies in reported photoluminescence quantum yields (PLQY) of this compound-doped phosphors?
- Methodology : Systematically vary dopant concentrations (1–5 mol%) and annealing atmospheres (N₂ vs. H₂/N₂). Use integrating spheres for absolute PLQY measurements.
- Contradiction Resolution : Identify surface oxidation (Tb³⁺ → Tb⁴⁺) as a quenching pathway via X-ray photoelectron spectroscopy (XPS) .
Q. Data Contradiction and Reproducibility
Q. Why do published values for this compound’s magnetic susceptibility vary across studies, and how can experimental protocols be standardized?
- Root Causes : Differences in sample hydration, grain size, or DC/AC measurement modes.
- Best Practices : Use SQUID magnetometry with in-situ drying (10⁻³ mbar, 24h) and report field-cooled/zero-field-cooled curves .
Q. What statistical models (e.g., Bayesian meta-analysis) are effective for reconciling conflicting solubility data of this compound in polar aprotic solvents?
- Approach : Aggregate datasets from literature, apply error-weighting, and model temperature-dependent solubility (ln S vs. 1/T) .
Q. Experimental Design Optimization
Q. How can fractional factorial design (FFD) optimize hydrothermal synthesis parameters for this compound nanoparticles?
- Variables : pH (2–6), precursor concentration (0.1–1.0 M), and reaction time (6–24h).
- Output Metrics : Particle size (TEM), colloidal stability (DLS), and phase purity (PXRD) .
Q. What in-situ monitoring techniques (e.g., Raman thermography) are critical for controlling this compound crystallization kinetics?
- Tools : Fiber-optic probes coupled with multivariate analysis (PLS regression) to track supersaturation and nucleation rates .
Q. Emerging Research Frontiers
Q. How do this compound-based electrolytes perform in solid-state batteries, and what interfacial challenges exist at Li-metal anodes?
Properties
CAS No. |
15162-98-8 |
---|---|
Molecular Formula |
Br3H2OTb |
Molecular Weight |
416.652 |
IUPAC Name |
terbium(3+);tribromide;hydrate |
InChI |
InChI=1S/3BrH.H2O.Tb/h3*1H;1H2;/q;;;;+3/p-3 |
InChI Key |
WQJPEPDSFBGXRP-UHFFFAOYSA-K |
SMILES |
O.[Br-].[Br-].[Br-].[Tb+3] |
Origin of Product |
United States |
Disclaimer and Information on In-Vitro Research Products
Please be aware that all articles and product information presented on BenchChem are intended solely for informational purposes. The products available for purchase on BenchChem are specifically designed for in-vitro studies, which are conducted outside of living organisms. In-vitro studies, derived from the Latin term "in glass," involve experiments performed in controlled laboratory settings using cells or tissues. It is important to note that these products are not categorized as medicines or drugs, and they have not received approval from the FDA for the prevention, treatment, or cure of any medical condition, ailment, or disease. We must emphasize that any form of bodily introduction of these products into humans or animals is strictly prohibited by law. It is essential to adhere to these guidelines to ensure compliance with legal and ethical standards in research and experimentation.