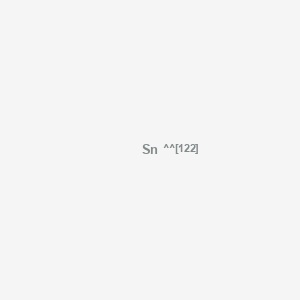
tin-122
- Click on QUICK INQUIRY to receive a quote from our team of experts.
- With the quality product at a COMPETITIVE price, you can focus more on your research.
Overview
Description
Tin-122 (¹²²Sn) is a stable isotope of tin, characterized by 50 protons and 72 neutrons . It accounts for 4.63% of natural tin abundance, making it the fifth most abundant stable tin isotope . Key properties include an atomic mass of 121.903439 Da, a body-centered tetragonal (BCT) or face-centered cubic (FCC) crystal structure (depending on allotrope), and a melting point of 505.08 K .
Preparation Methods
The preparation of tin-122 typically involves the separation of tin isotopes from naturally occurring tin. This can be achieved through various methods such as gas centrifugation or electromagnetic separation. Industrial production of this compound may involve the use of advanced techniques like laser isotope separation, which allows for the precise extraction of specific isotopes from a mixture .
Chemical Reactions Analysis
Tin-122, like other tin isotopes, can undergo various chemical reactions. Some of the common types of reactions include:
Oxidation: Tin can react with oxygen to form tin oxides. For example, when heated in the presence of oxygen, tin can form tin(IV) oxide (SnO₂).
Reduction: Tin can be reduced from its oxides using reducing agents like hydrogen or carbon.
Substitution: Tin can participate in substitution reactions, where it replaces another element in a compound. .
Scientific Research Applications
Tin-122 has several applications in scientific research, including:
Chemistry: this compound is used in the study of tin compounds and their reactions. It is also used in the synthesis of various tin-based materials.
Biology: Tin compounds, including those containing this compound, are studied for their potential biological activities, such as antimicrobial properties.
Medicine: this compound is used in the development of radiopharmaceuticals for medical imaging and diagnostic purposes.
Industry: This compound is used in the production of tin-based alloys and coatings, which are valued for their corrosion resistance and other desirable properties .
Mechanism of Action
The mechanism of action of tin-122 depends on the specific compound it is part of. For example, in radiopharmaceuticals, this compound can act as a reducing agent, facilitating the formation of complexes with other elements. These complexes can then be used for imaging or therapeutic purposes. The molecular targets and pathways involved vary depending on the specific application and compound .
Comparison with Similar Compounds
Comparison with Other Stable Tin Isotopes
Tin has ten stable isotopes, with tin-120 (32.59% abundance) and tin-124 (5.8% abundance) being the closest in mass to tin-122. Table 1 highlights their comparative properties:
Table 1: Comparative Properties of Tin-120, this compound, and Tin-124
Property | Tin-120 | This compound | Tin-124 |
---|---|---|---|
Natural Abundance (%) | 32.59 | 4.63 | 5.8 |
Neutron Number | 70 | 72 | 74 |
Formation Process | s-process | s-process | r-process |
Half-Life | Stable | Stable | Stable |
Key Differences:
- Nucleosynthesis: Tin-120 and this compound are primarily formed via the slow neutron capture (s-process) in low-to-medium mass stars, whereas tin-124 originates from rapid neutron capture (r-process) in supernovae or neutron star mergers .
- Neutron Capture Cross-Section : this compound has a lower neutron capture probability compared to tin-124, influencing its abundance in stellar environments .
Comparison with A=122 Isobars
This compound belongs to the isobaric series with mass number 122, which includes antimony-122 (¹²²Sb) and tellurium-122 (¹²²Te). Table 2 summarizes their nuclear characteristics:
Table 2: A=122 Isobars Comparison
Nuclear Stability Insights :
- This compound and tellurium-122 are both stable, whereas antimony-122 is unstable due to its imbalanced proton-neutron ratio. The decay energy of ¹²²Sb favors β⁻ decay to ¹²²Te over β⁺/electron capture to ¹²²Sn by 0.37 MeV .
Production and Availability
This compound is produced in smaller quantities compared to more abundant isotopes like tin-120. At the Y-12 National Security Complex, documented production batches include 150 mg and 20 mg of this compound, significantly less than tin-120 (837 mg) or tin-118 (1,790 mg) .
Q & A
Basic Research Questions
Q. What are the key nuclear properties of tin-122, and how do they influence its stability compared to other tin isotopes?
this compound (Sn-122) is a stable isotope with a natural isotopic abundance influenced by its nuclear shell structure. Its stability is notable in the context of neighboring isobars like antimony-122 (Sb-122) and tellurium-122 (Te-122), which exhibit dual decay pathways due to mass differences (Sb-122 can decay into Te-122 or Sn-122 via β⁻ or β⁺ decay, respectively) . Key properties include its atomic mass (121.9034 u) and its role in studying double beta decay processes. Researchers should prioritize mass spectrometry and gamma spectroscopy to characterize its isotopic purity and decay products .
Q. What methodologies are recommended for synthesizing and isolating this compound in laboratory settings?
this compound can be produced via nuclear reactions or isotopic separation. Evidence from production data indicates yields of 150 mg ± 20 mg, highlighting the need for high-resolution electromagnetic separation to minimize contamination from other tin isotopes (e.g., Sn-120, Sn-124) . Purification protocols should include chemical etching to remove surface oxides and neutron activation analysis to validate isotopic composition. Cross-referencing with databases like the National Nuclear Data Center ensures consistency in reported yields .
Advanced Research Questions
Q. How do theoretical models reconcile the stability of this compound with its potential for double beta decay?
While this compound is classified as stable, its position in the chart of nuclides suggests proximity to potential double beta decay candidates (e.g., Te-128). Theoretical frameworks like the nuclear shell model and Hartree-Fock calculations predict Sn-122's stability by analyzing binding energy differences with adjacent isobars. Researchers should compare computed decay energies (e.g., Sb-122 → Sn-122 releases 1.61 MeV) against experimental measurements to validate models . Monte Carlo simulations can further assess decay probabilities under varying nuclear configurations.
Q. What experimental challenges arise in measuring this compound’s isotopic abundance in complex matrices, and how can they be mitigated?
Challenges include interference from isobaric contaminants (e.g., Cd-122, Te-122) and matrix effects in mass spectrometry. Methodological solutions involve using tandem mass spectrometers with collision/reaction cells to suppress polyatomic interferences. Additionally, isotopic dilution analysis with enriched Sn-122 tracers improves quantification accuracy. Researchers must report uncertainty margins (e.g., ±20 mg as per production data) and validate results against certified reference materials .
Q. How can contradictions in reported decay energies or isotopic abundances of this compound be resolved?
Discrepancies often stem from methodological variability, such as differences in detector calibration or sample preparation. A systematic approach includes:
- Replicating experiments using standardized protocols (e.g., IAEA guidelines for isotopic measurements).
- Applying meta-analysis to aggregate data from multiple studies, weighting results by measurement precision.
- Utilizing ab-initio nuclear models to identify outliers in decay energy datasets . Cross-disciplinary collaboration with nuclear physicists and radiochemists is critical to resolving such contradictions .
Q. Methodological Guidance
Q. What analytical techniques are most effective for characterizing this compound in environmental or astrophysical samples?
- Neutron Activation Analysis (NAA): Detects trace Sn-122 via neutron capture reactions, followed by gamma-ray spectroscopy.
- Secondary Ion Mass Spectrometry (SIMS): Provides spatially resolved isotopic maps, ideal for meteoritic or geological samples.
- Collinear Laser Spectroscopy: Measures hyperfine structure to determine nuclear spin and charge radius differences . Ensure calibration with isotopically enriched standards and document detection limits in publications .
Q. How should researchers design experiments to investigate this compound’s role in nucleosynthesis or nuclear astrophysics?
Experimental design should:
- Simulate astrophysical environments (e.g., s-process conditions) using particle accelerators to study neutron capture cross-sections.
- Incorporate isotopic tracers (e.g., Sn-122 enriched precursors) in reaction networks.
- Compare results with observational data from stellar spectra or presolar grain analyses . Peer-review protocols recommend pre-registering experimental parameters to enhance reproducibility .
Q. Data Presentation and Publishing Standards
Properties
CAS No. |
14119-18-7 |
---|---|
Molecular Formula |
Sn |
Molecular Weight |
121.903 |
IUPAC Name |
tin-122 |
InChI |
InChI=1S/Sn/i1+3 |
InChI Key |
ATJFFYVFTNAWJD-AKLPVKDBSA-N |
SMILES |
[Sn] |
Origin of Product |
United States |
Disclaimer and Information on In-Vitro Research Products
Please be aware that all articles and product information presented on BenchChem are intended solely for informational purposes. The products available for purchase on BenchChem are specifically designed for in-vitro studies, which are conducted outside of living organisms. In-vitro studies, derived from the Latin term "in glass," involve experiments performed in controlled laboratory settings using cells or tissues. It is important to note that these products are not categorized as medicines or drugs, and they have not received approval from the FDA for the prevention, treatment, or cure of any medical condition, ailment, or disease. We must emphasize that any form of bodily introduction of these products into humans or animals is strictly prohibited by law. It is essential to adhere to these guidelines to ensure compliance with legal and ethical standards in research and experimentation.