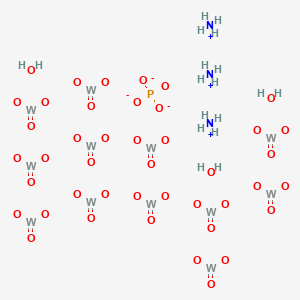
Ammonium 12-tungstophosphate
- Click on QUICK INQUIRY to receive a quote from our team of experts.
- With the quality product at a COMPETITIVE price, you can focus more on your research.
Overview
Description
Ammonium 12-tungstophosphate is a heteropoly acid salt with the molecular formula (NH₄)₃PW₁₂O₄₀. It is known for its high molecular weight and complex structure, which includes twelve tungsten atoms coordinated around a central phosphate group. This compound is notable for its strong acidic properties and high thermal stability, making it useful in various scientific and industrial applications .
Preparation Methods
Synthetic Routes and Reaction Conditions: Ammonium 12-tungstophosphate can be synthesized through the reaction of phosphoric acid with ammonium tungstate under controlled conditions. The typical reaction involves dissolving ammonium tungstate in water, followed by the addition of phosphoric acid. The mixture is then heated to promote the formation of the heteropoly acid salt. The reaction conditions, such as temperature and pH, are carefully controlled to ensure the purity and yield of the product .
Industrial Production Methods: In industrial settings, the production of this compound involves large-scale reactors where the reactants are mixed and heated under controlled conditions. The product is then purified through crystallization and filtration processes. The industrial methods focus on optimizing the yield and purity while minimizing the production costs .
Chemical Reactions Analysis
Types of Reactions: Ammonium 12-tungstophosphate undergoes various chemical reactions, including:
Oxidation: It can act as an oxidizing agent in certain reactions.
Reduction: It can be reduced under specific conditions to form lower oxidation state compounds.
Substitution: It can participate in substitution reactions where one or more of its components are replaced by other atoms or groups
Common Reagents and Conditions:
Oxidation: Common oxidizing agents include hydrogen peroxide and potassium permanganate.
Reduction: Reducing agents such as hydrogen gas or hydrazine can be used.
Substitution: Various organic and inorganic reagents can be used depending on the desired substitution.
Major Products: The major products formed from these reactions depend on the specific conditions and reagents used. For example, oxidation reactions may produce tungsten oxides, while reduction reactions may yield lower oxidation state tungsten compounds .
Scientific Research Applications
Ammonium 12-tungstophosphate has a wide range of applications in scientific research:
Mechanism of Action
The mechanism by which ammonium 12-tungstophosphate exerts its effects is primarily through its strong acidic properties and ability to act as a catalyst. It can facilitate various chemical reactions by providing a suitable environment for the reactants to interact. The molecular targets and pathways involved depend on the specific application and reaction conditions .
Comparison with Similar Compounds
Phosphotungstic Acid: Similar in structure but differs in its protonation state and solubility properties.
Ammonium Molybdophosphate: Similar in its use as an ion-exchange material but differs in its metal center (molybdenum instead of tungsten).
Uniqueness: Ammonium 12-tungstophosphate is unique due to its high thermal stability and strong acidic properties, which make it an effective catalyst in various chemical reactions. Its ability to form stable complexes with various ions and molecules also sets it apart from similar compounds .
Properties
IUPAC Name |
triazanium;trioxotungsten;phosphate;trihydrate |
Source
|
---|---|---|
Source | PubChem | |
URL | https://pubchem.ncbi.nlm.nih.gov | |
Description | Data deposited in or computed by PubChem | |
InChI |
InChI=1S/3H3N.H3O4P.3H2O.36O.12W/c;;;1-5(2,3)4;;;;;;;;;;;;;;;;;;;;;;;;;;;;;;;;;;;;;;;;;;;;;;;;;;;/h3*1H3;(H3,1,2,3,4);3*1H2;;;;;;;;;;;;;;;;;;;;;;;;;;;;;;;;;;;;;;;;;;;;;;;; |
Source
|
Source | PubChem | |
URL | https://pubchem.ncbi.nlm.nih.gov | |
Description | Data deposited in or computed by PubChem | |
InChI Key |
KMHQDGHAAPSFGS-UHFFFAOYSA-N |
Source
|
Source | PubChem | |
URL | https://pubchem.ncbi.nlm.nih.gov | |
Description | Data deposited in or computed by PubChem | |
Canonical SMILES |
[NH4+].[NH4+].[NH4+].O.O.O.[O-]P(=O)([O-])[O-].O=[W](=O)=O.O=[W](=O)=O.O=[W](=O)=O.O=[W](=O)=O.O=[W](=O)=O.O=[W](=O)=O.O=[W](=O)=O.O=[W](=O)=O.O=[W](=O)=O.O=[W](=O)=O.O=[W](=O)=O.O=[W](=O)=O |
Source
|
Source | PubChem | |
URL | https://pubchem.ncbi.nlm.nih.gov | |
Description | Data deposited in or computed by PubChem | |
Molecular Formula |
H18N3O43PW12 |
Source
|
Source | PubChem | |
URL | https://pubchem.ncbi.nlm.nih.gov | |
Description | Data deposited in or computed by PubChem | |
Molecular Weight |
2985.2 g/mol |
Source
|
Source | PubChem | |
URL | https://pubchem.ncbi.nlm.nih.gov | |
Description | Data deposited in or computed by PubChem | |
CAS No. |
1311-90-6 |
Source
|
Record name | Ammonium 12-tungstophosphate | |
Source | ChemIDplus | |
URL | https://pubchem.ncbi.nlm.nih.gov/substance/?source=chemidplus&sourceid=0001311906 | |
Description | ChemIDplus is a free, web search system that provides access to the structure and nomenclature authority files used for the identification of chemical substances cited in National Library of Medicine (NLM) databases, including the TOXNET system. | |
Record name | AMMONIUM PHOSPHOTUNGSTATE TRIHYDRATE | |
Source | FDA Global Substance Registration System (GSRS) | |
URL | https://gsrs.ncats.nih.gov/ginas/app/beta/substances/IE58999G9P | |
Description | The FDA Global Substance Registration System (GSRS) enables the efficient and accurate exchange of information on what substances are in regulated products. Instead of relying on names, which vary across regulatory domains, countries, and regions, the GSRS knowledge base makes it possible for substances to be defined by standardized, scientific descriptions. | |
Explanation | Unless otherwise noted, the contents of the FDA website (www.fda.gov), both text and graphics, are not copyrighted. They are in the public domain and may be republished, reprinted and otherwise used freely by anyone without the need to obtain permission from FDA. Credit to the U.S. Food and Drug Administration as the source is appreciated but not required. | |
Disclaimer and Information on In-Vitro Research Products
Please be aware that all articles and product information presented on BenchChem are intended solely for informational purposes. The products available for purchase on BenchChem are specifically designed for in-vitro studies, which are conducted outside of living organisms. In-vitro studies, derived from the Latin term "in glass," involve experiments performed in controlled laboratory settings using cells or tissues. It is important to note that these products are not categorized as medicines or drugs, and they have not received approval from the FDA for the prevention, treatment, or cure of any medical condition, ailment, or disease. We must emphasize that any form of bodily introduction of these products into humans or animals is strictly prohibited by law. It is essential to adhere to these guidelines to ensure compliance with legal and ethical standards in research and experimentation.