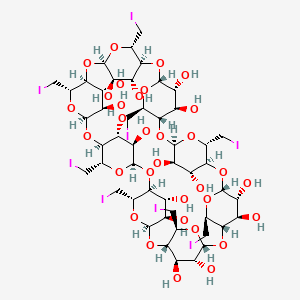
Octakis-6-iodo-6-deoxy-gamma-cyclodextrin
- Click on QUICK INQUIRY to receive a quote from our team of experts.
- With the quality product at a COMPETITIVE price, you can focus more on your research.
Overview
Description
Octakis-6-iodo-6-deoxy-gamma-cyclodextrin: is a chemically modified cyclodextrin, where eight iodine atoms replace the hydroxyl groups at the sixth position of the glucose units in gamma-cyclodextrin. This modification enhances its chemical properties, making it useful in various scientific and industrial applications .
Preparation Methods
Synthetic Routes and Reaction Conditions: The synthesis of Octakis-6-iodo-6-deoxy-gamma-cyclodextrin involves the iodination of gamma-cyclodextrin. The process typically includes the following steps:
Activation of Gamma-Cyclodextrin: Gamma-cyclodextrin is first activated by converting the hydroxyl groups at the sixth position to a leaving group, such as a tosylate.
Iodination: The activated gamma-cyclodextrin is then reacted with sodium iodide in the presence of a suitable solvent like dimethylformamide (DMF) to replace the leaving groups with iodine atoms
Industrial Production Methods: Industrial production of this compound follows similar synthetic routes but on a larger scale. The process is optimized for higher yields and purity, often involving advanced purification techniques like high-performance liquid chromatography (HPLC) to ensure the removal of impurities .
Chemical Reactions Analysis
Types of Reactions: Octakis-6-iodo-6-deoxy-gamma-cyclodextrin undergoes various chemical reactions, including:
Substitution Reactions: The iodine atoms can be replaced with other functional groups through nucleophilic substitution reactions.
Oxidation and Reduction: The compound can participate in redox reactions, where the iodine atoms can be oxidized or reduced under specific conditions
Common Reagents and Conditions:
Nucleophilic Substitution: Common reagents include sodium azide, thiourea, and other nucleophiles. The reactions are typically carried out in polar aprotic solvents like DMF or dimethyl sulfoxide (DMSO).
Oxidation and Reduction: Reagents such as hydrogen peroxide or sodium borohydride are used for oxidation and reduction reactions, respectively
Major Products: The major products formed from these reactions depend on the reagents used. For example, substitution with sodium azide yields azido-functionalized cyclodextrins, while reduction with sodium borohydride produces deiodinated derivatives .
Scientific Research Applications
Chemistry: Octakis-6-iodo-6-deoxy-gamma-cyclodextrin is used as a precursor for synthesizing various functionalized cyclodextrins.
Biology and Medicine: In biological research, this compound is used to study host-guest interactions and drug delivery systems. Its ability to form inclusion complexes with various molecules makes it a potential candidate for enhancing the solubility and stability of pharmaceuticals .
Industry: Industrially, this compound is employed in the development of advanced materials, such as sensors and catalysts. Its unique properties enable the creation of materials with specific functionalities .
Mechanism of Action
The mechanism by which Octakis-6-iodo-6-deoxy-gamma-cyclodextrin exerts its effects is primarily through its ability to form inclusion complexes. The iodine atoms enhance its binding affinity for various guest molecules, facilitating their encapsulation within the cyclodextrin cavity. This property is exploited in drug delivery systems to improve the bioavailability and stability of therapeutic agents .
Comparison with Similar Compounds
Gamma-Cyclodextrin: The parent compound, gamma-cyclodextrin, lacks the iodine modifications and has different solubility and binding properties.
Octakis-6-deoxy-6-chloro-gamma-cyclodextrin: Similar to the iodo derivative but with chlorine atoms, offering different reactivity and applications.
Octakis-6-deoxy-6-bromo-gamma-cyclodextrin: Bromine-substituted derivative with distinct chemical properties
Uniqueness: Octakis-6-iodo-6-deoxy-gamma-cyclodextrin is unique due to the presence of iodine atoms, which impart specific chemical reactivity and binding characteristics. This makes it particularly useful in applications requiring strong host-guest interactions and specific functionalization .
Properties
IUPAC Name |
(1S,3S,5S,6S,8S,10S,11S,13S,15S,16S,18S,20S,21S,23S,25S,26S,28S,30S,31S,33S,35S,36S,38S,40S,41R,42R,43R,44R,45R,46R,47R,48R,49R,50R,51R,52R,53R,54R,55R,56R)-5,10,15,20,25,30,35,40-octakis(iodomethyl)-2,4,7,9,12,14,17,19,22,24,27,29,32,34,37,39-hexadecaoxanonacyclo[36.2.2.23,6.28,11.213,16.218,21.223,26.228,31.233,36]hexapentacontane-41,42,43,44,45,46,47,48,49,50,51,52,53,54,55,56-hexadecol |
Source
|
---|---|---|
Source | PubChem | |
URL | https://pubchem.ncbi.nlm.nih.gov | |
Description | Data deposited in or computed by PubChem | |
InChI |
InChI=1S/C48H72I8O32/c49-1-9-33-17(57)25(65)41(73-9)82-34-10(2-50)75-43(27(67)19(34)59)84-36-12(4-52)77-45(29(69)21(36)61)86-38-14(6-54)79-47(31(71)23(38)63)88-40-16(8-56)80-48(32(72)24(40)64)87-39-15(7-55)78-46(30(70)22(39)62)85-37-13(5-53)76-44(28(68)20(37)60)83-35-11(3-51)74-42(81-33)26(66)18(35)58/h9-48,57-72H,1-8H2/t9-,10-,11-,12-,13-,14-,15-,16-,17-,18-,19-,20-,21-,22-,23-,24-,25-,26-,27-,28-,29-,30-,31-,32-,33-,34-,35-,36-,37-,38-,39-,40-,41-,42-,43-,44-,45-,46-,47-,48-/m1/s1 |
Source
|
Source | PubChem | |
URL | https://pubchem.ncbi.nlm.nih.gov | |
Description | Data deposited in or computed by PubChem | |
InChI Key |
IHISFVNFOGFWNA-HSEONFRVSA-N |
Source
|
Source | PubChem | |
URL | https://pubchem.ncbi.nlm.nih.gov | |
Description | Data deposited in or computed by PubChem | |
Canonical SMILES |
C(C1C2C(C(C(O1)OC3C(OC(C(C3O)O)OC4C(OC(C(C4O)O)OC5C(OC(C(C5O)O)OC6C(OC(C(C6O)O)OC7C(OC(C(C7O)O)OC8C(OC(C(C8O)O)OC9C(OC(O2)C(C9O)O)CI)CI)CI)CI)CI)CI)CI)O)O)I |
Source
|
Source | PubChem | |
URL | https://pubchem.ncbi.nlm.nih.gov | |
Description | Data deposited in or computed by PubChem | |
Isomeric SMILES |
C([C@@H]1[C@@H]2[C@@H]([C@H]([C@H](O1)O[C@@H]3[C@H](O[C@@H]([C@@H]([C@H]3O)O)O[C@@H]4[C@H](O[C@@H]([C@@H]([C@H]4O)O)O[C@@H]5[C@H](O[C@@H]([C@@H]([C@H]5O)O)O[C@@H]6[C@H](O[C@@H]([C@@H]([C@H]6O)O)O[C@@H]7[C@H](O[C@@H]([C@@H]([C@H]7O)O)O[C@@H]8[C@H](O[C@@H]([C@@H]([C@H]8O)O)O[C@@H]9[C@H](O[C@H](O2)[C@@H]([C@H]9O)O)CI)CI)CI)CI)CI)CI)CI)O)O)I |
Source
|
Source | PubChem | |
URL | https://pubchem.ncbi.nlm.nih.gov | |
Description | Data deposited in or computed by PubChem | |
Molecular Formula |
C48H72I8O32 |
Source
|
Source | PubChem | |
URL | https://pubchem.ncbi.nlm.nih.gov | |
Description | Data deposited in or computed by PubChem | |
Molecular Weight |
2176.3 g/mol |
Source
|
Source | PubChem | |
URL | https://pubchem.ncbi.nlm.nih.gov | |
Description | Data deposited in or computed by PubChem | |
Disclaimer and Information on In-Vitro Research Products
Please be aware that all articles and product information presented on BenchChem are intended solely for informational purposes. The products available for purchase on BenchChem are specifically designed for in-vitro studies, which are conducted outside of living organisms. In-vitro studies, derived from the Latin term "in glass," involve experiments performed in controlled laboratory settings using cells or tissues. It is important to note that these products are not categorized as medicines or drugs, and they have not received approval from the FDA for the prevention, treatment, or cure of any medical condition, ailment, or disease. We must emphasize that any form of bodily introduction of these products into humans or animals is strictly prohibited by law. It is essential to adhere to these guidelines to ensure compliance with legal and ethical standards in research and experimentation.