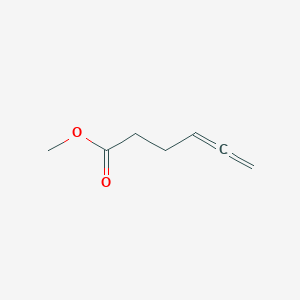
Methyl hexa-4,5-dienoate
Overview
Description
Methyl hexa-4,5-dienoate is an organic compound with the molecular formula C7H10O2. It is a derivative of hexa-4,5-dienoic acid, where the carboxylic acid group is esterified with methanol. This compound is characterized by its conjugated diene system, which consists of two double bonds separated by a single bond, making it a conjugated diene ester.
Preparation Methods
Synthetic Routes and Reaction Conditions
Methyl hexa-4,5-dienoate can be synthesized through the esterification of hexa-4,5-dienoic acid with methanol. This reaction typically requires an acid catalyst, such as sulfuric acid or p-toluenesulfonic acid, to proceed efficiently. The reaction is carried out under reflux conditions to ensure complete conversion of the acid to the ester.
Industrial Production Methods
In an industrial setting, the production of this compound may involve continuous esterification processes. These processes utilize large-scale reactors where hexa-4,5-dienoic acid and methanol are continuously fed into the reactor, and the ester product is continuously removed. The use of solid acid catalysts, such as ion-exchange resins, can enhance the efficiency and sustainability of the process.
Chemical Reactions Analysis
Types of Reactions
Methyl hexa-4,5-dienoate undergoes various chemical reactions, including:
Oxidation: The compound can be oxidized to form corresponding carboxylic acids or aldehydes.
Reduction: Reduction reactions can convert the double bonds into single bonds, yielding saturated esters.
Substitution: The ester group can undergo nucleophilic substitution reactions, where the methoxy group is replaced by other nucleophiles.
Common Reagents and Conditions
Oxidation: Common oxidizing agents include potassium permanganate (KMnO4) and chromium trioxide (CrO3).
Reduction: Catalytic hydrogenation using palladium on carbon (Pd/C) or lithium aluminum hydride (LiAlH4) can be employed.
Substitution: Nucleophiles such as amines or thiols can be used under basic conditions to achieve substitution reactions.
Major Products Formed
Oxidation: Hexa-4,5-dienoic acid or hexa-4,5-dienal.
Reduction: Methyl hexanoate.
Substitution: Various substituted esters depending on the nucleophile used.
Scientific Research Applications
Methyl hexa-4,5-dienoate has several applications in scientific research:
Chemistry: It is used as a building block in organic synthesis, particularly in the synthesis of more complex molecules.
Biology: The compound can be used in studies involving enzyme-catalyzed reactions, especially those involving esterases and lipases.
Medicine: Research into potential pharmaceutical applications, such as drug delivery systems, where the ester functionality can be exploited for controlled release.
Industry: Utilized in the production of fragrances and flavors due to its ester group, which imparts pleasant aromas.
Mechanism of Action
The mechanism of action of methyl hexa-4,5-dienoate in biological systems involves its interaction with enzymes that catalyze ester hydrolysis. Esterases and lipases can hydrolyze the ester bond, releasing hexa-4,5-dienoic acid and methanol. The conjugated diene system can also participate in various biochemical pathways, potentially interacting with cellular receptors and enzymes involved in oxidative stress responses.
Comparison with Similar Compounds
Similar Compounds
Methyl sorbate: Another ester of sorbic acid, with similar conjugated diene structure.
Methyl hexanoate: A saturated ester with no conjugated double bonds.
Ethyl hexa-4,5-dienoate: An ester with an ethyl group instead of a methyl group.
Uniqueness
Methyl hexa-4,5-dienoate is unique due to its specific conjugated diene system, which imparts distinct chemical reactivity and potential biological activity. Its ability to undergo various chemical transformations makes it a versatile compound in synthetic chemistry and industrial applications.
Biological Activity
Methyl hexa-4,5-dienoate, also known as methyl 5-methylhexa-2,5-dienoate, is a compound of significant interest due to its unique structural features and potential biological activities. This article explores its biological activity, mechanisms of action, and relevant research findings.
Chemical Structure and Properties
This compound is characterized by its molecular formula and a molecular weight of approximately 140.18 g/mol. The compound features a conjugated diene system with alternating double bonds, which enhances its reactivity and potential interactions with biological targets.
The biological activity of this compound is primarily attributed to its ability to engage in electron transfer reactions facilitated by its conjugated diene system. This reactivity allows the compound to modulate various biochemical pathways, potentially influencing enzyme activity and receptor interactions. Research suggests that this compound may exhibit anti-inflammatory and antimicrobial properties through these mechanisms.
Anti-inflammatory Effects
Studies have indicated that this compound can modulate inflammatory responses. It has been shown to inhibit the production of pro-inflammatory cytokines in various cell lines, suggesting a potential therapeutic role in treating inflammatory diseases. This effect may be mediated through the inhibition of specific signaling pathways involved in inflammation.
Antimicrobial Properties
Research has demonstrated that this compound exhibits antimicrobial activity against a range of pathogens. Its efficacy against both gram-positive and gram-negative bacteria highlights its potential as a natural preservative or therapeutic agent. The exact mechanism behind this antimicrobial action is believed to involve disruption of microbial cell membranes due to the compound's lipophilic nature.
Table 1: Summary of Biological Activities
Activity | Effect | Mechanism |
---|---|---|
Anti-inflammatory | Inhibition of cytokine production | Modulation of signaling pathways |
Antimicrobial | Activity against bacteria | Disruption of cell membranes |
Case Study: Anti-inflammatory Effects
A study conducted on human macrophage cell lines demonstrated that treatment with this compound resulted in a significant reduction in the secretion of TNF-alpha and IL-6, key cytokines involved in the inflammatory response. The compound was administered at varying concentrations (10 µM to 100 µM), with the most pronounced effects observed at 50 µM.
Case Study: Antimicrobial Efficacy
In another investigation, this compound was tested against Staphylococcus aureus and Escherichia coli. The minimum inhibitory concentration (MIC) was found to be 32 µg/mL for S. aureus and 64 µg/mL for E. coli, indicating moderate antimicrobial potency. The study concluded that the compound could serve as a potential candidate for developing new antimicrobial agents.
Properties
InChI |
InChI=1S/C7H10O2/c1-3-4-5-6-7(8)9-2/h4H,1,5-6H2,2H3 | |
---|---|---|
Source | PubChem | |
URL | https://pubchem.ncbi.nlm.nih.gov | |
Description | Data deposited in or computed by PubChem | |
InChI Key |
CUEIYTRWDOPVOE-UHFFFAOYSA-N | |
Source | PubChem | |
URL | https://pubchem.ncbi.nlm.nih.gov | |
Description | Data deposited in or computed by PubChem | |
Canonical SMILES |
COC(=O)CCC=C=C | |
Source | PubChem | |
URL | https://pubchem.ncbi.nlm.nih.gov | |
Description | Data deposited in or computed by PubChem | |
Molecular Formula |
C7H10O2 | |
Source | PubChem | |
URL | https://pubchem.ncbi.nlm.nih.gov | |
Description | Data deposited in or computed by PubChem | |
DSSTOX Substance ID |
DTXSID30628493 | |
Record name | Methyl hexa-4,5-dienoate | |
Source | EPA DSSTox | |
URL | https://comptox.epa.gov/dashboard/DTXSID30628493 | |
Description | DSSTox provides a high quality public chemistry resource for supporting improved predictive toxicology. | |
Molecular Weight |
126.15 g/mol | |
Source | PubChem | |
URL | https://pubchem.ncbi.nlm.nih.gov | |
Description | Data deposited in or computed by PubChem | |
CAS No. |
114971-88-9 | |
Record name | Methyl hexa-4,5-dienoate | |
Source | EPA DSSTox | |
URL | https://comptox.epa.gov/dashboard/DTXSID30628493 | |
Description | DSSTox provides a high quality public chemistry resource for supporting improved predictive toxicology. | |
Retrosynthesis Analysis
AI-Powered Synthesis Planning: Our tool employs the Template_relevance Pistachio, Template_relevance Bkms_metabolic, Template_relevance Pistachio_ringbreaker, Template_relevance Reaxys, Template_relevance Reaxys_biocatalysis model, leveraging a vast database of chemical reactions to predict feasible synthetic routes.
One-Step Synthesis Focus: Specifically designed for one-step synthesis, it provides concise and direct routes for your target compounds, streamlining the synthesis process.
Accurate Predictions: Utilizing the extensive PISTACHIO, BKMS_METABOLIC, PISTACHIO_RINGBREAKER, REAXYS, REAXYS_BIOCATALYSIS database, our tool offers high-accuracy predictions, reflecting the latest in chemical research and data.
Strategy Settings
Precursor scoring | Relevance Heuristic |
---|---|
Min. plausibility | 0.01 |
Model | Template_relevance |
Template Set | Pistachio/Bkms_metabolic/Pistachio_ringbreaker/Reaxys/Reaxys_biocatalysis |
Top-N result to add to graph | 6 |
Feasible Synthetic Routes
Disclaimer and Information on In-Vitro Research Products
Please be aware that all articles and product information presented on BenchChem are intended solely for informational purposes. The products available for purchase on BenchChem are specifically designed for in-vitro studies, which are conducted outside of living organisms. In-vitro studies, derived from the Latin term "in glass," involve experiments performed in controlled laboratory settings using cells or tissues. It is important to note that these products are not categorized as medicines or drugs, and they have not received approval from the FDA for the prevention, treatment, or cure of any medical condition, ailment, or disease. We must emphasize that any form of bodily introduction of these products into humans or animals is strictly prohibited by law. It is essential to adhere to these guidelines to ensure compliance with legal and ethical standards in research and experimentation.