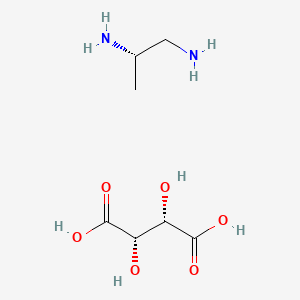
(S)-(-)-Diaminopropane tartaric acid
Overview
Description
(S)-(-)-Diaminopropane tartaric acid is a chiral compound that combines the properties of diaminopropane and tartaric acid
Preparation Methods
Synthetic Routes and Reaction Conditions
The preparation of (S)-(-)-Diaminopropane tartaric acid typically involves the resolution of racemic mixtures using chiral reagents. One common method is the reaction of racemic diaminopropane with enantiomerically pure tartaric acid to form diastereomeric salts, which can then be separated based on their differing physical properties . Another approach involves the use of penicillin molds that selectively consume one enantiomer, leaving the desired this compound .
Industrial Production Methods
Industrial production of this compound often relies on large-scale resolution techniques. These methods include the use of chiral chromatography or crystallization techniques to separate the desired enantiomer from a racemic mixture. The process may also involve the use of specific molds or bacteria that preferentially metabolize one enantiomer, allowing for the isolation of the other .
Chemical Reactions Analysis
Types of Reactions
(S)-(-)-Diaminopropane tartaric acid can undergo various chemical reactions, including:
Oxidation: It can be oxidized to form dioxosuccinic acid using reagents like hydrogen peroxide and ferrous sulfate.
Reduction: Reduction with hydriodic acid yields succinic acid.
Substitution: It can react with ammonio-silver nitrate to produce metallic silver.
Common Reagents and Conditions
Common reagents used in these reactions include hydrogen peroxide, ferrous sulfate, hydriodic acid, and ammonio-silver nitrate. The conditions for these reactions typically involve controlled temperatures and specific pH levels to ensure the desired transformations .
Major Products
The major products formed from these reactions include dioxosuccinic acid, succinic acid, and metallic silver, depending on the specific reaction conditions and reagents used .
Scientific Research Applications
(S)-(-)-Diaminopropane tartaric acid has a wide range of applications in scientific research:
Mechanism of Action
The mechanism of action of (S)-(-)-Diaminopropane tartaric acid involves its interaction with specific molecular targets and pathways. For instance, it acts as a muscle toxin by inhibiting the production of malic acid, which can lead to paralysis in high doses . The compound’s chiral nature allows it to interact selectively with various enzymes and proteins, influencing biochemical pathways and cellular functions .
Comparison with Similar Compounds
Similar Compounds
(R,R)-Tartaric acid: The enantiomer of (S,S)-tartaric acid, with similar physical properties but opposite optical rotation.
Meso-tartaric acid: An optically inactive form of tartaric acid with distinct physical properties compared to the enantiomerically pure forms.
Succinic acid: A structurally related compound that shares some chemical properties but lacks the chiral centers present in tartaric acid.
Uniqueness
(S)-(-)-Diaminopropane tartaric acid is unique due to its combination of diaminopropane and tartaric acid moieties, providing both chiral centers and functional groups that enable a wide range of chemical reactions and applications. Its ability to act as a resolving agent and its specific interactions with biological molecules make it particularly valuable in research and industrial applications .
Properties
IUPAC Name |
(2S,3S)-2,3-dihydroxybutanedioic acid;(2S)-propane-1,2-diamine | |
---|---|---|
Source | PubChem | |
URL | https://pubchem.ncbi.nlm.nih.gov | |
Description | Data deposited in or computed by PubChem | |
InChI |
InChI=1S/C4H6O6.C3H10N2/c5-1(3(7)8)2(6)4(9)10;1-3(5)2-4/h1-2,5-6H,(H,7,8)(H,9,10);3H,2,4-5H2,1H3/t1-,2-;3-/m00/s1 | |
Source | PubChem | |
URL | https://pubchem.ncbi.nlm.nih.gov | |
Description | Data deposited in or computed by PubChem | |
InChI Key |
GMEKUQIZTKWBJU-KBZAOUMQSA-N | |
Source | PubChem | |
URL | https://pubchem.ncbi.nlm.nih.gov | |
Description | Data deposited in or computed by PubChem | |
Canonical SMILES |
CC(CN)N.C(C(C(=O)O)O)(C(=O)O)O | |
Source | PubChem | |
URL | https://pubchem.ncbi.nlm.nih.gov | |
Description | Data deposited in or computed by PubChem | |
Isomeric SMILES |
C[C@@H](CN)N.[C@H]([C@@H](C(=O)O)O)(C(=O)O)O | |
Source | PubChem | |
URL | https://pubchem.ncbi.nlm.nih.gov | |
Description | Data deposited in or computed by PubChem | |
Molecular Formula |
C7H16N2O6 | |
Source | PubChem | |
URL | https://pubchem.ncbi.nlm.nih.gov | |
Description | Data deposited in or computed by PubChem | |
Related CAS |
58649-86-8 | |
Record name | 1,2-Propanediamine, (2S)-, (2S,3S)-2,3-dihydroxybutanedioate (1:2) | |
Source | CAS Common Chemistry | |
URL | https://commonchemistry.cas.org/detail?cas_rn=58649-86-8 | |
Description | CAS Common Chemistry is an open community resource for accessing chemical information. Nearly 500,000 chemical substances from CAS REGISTRY cover areas of community interest, including common and frequently regulated chemicals, and those relevant to high school and undergraduate chemistry classes. This chemical information, curated by our expert scientists, is provided in alignment with our mission as a division of the American Chemical Society. | |
Explanation | The data from CAS Common Chemistry is provided under a CC-BY-NC 4.0 license, unless otherwise stated. | |
DSSTOX Substance ID |
DTXSID90669968 | |
Record name | (2S,3S)-2,3-Dihydroxybutanedioic acid--(2S)-propane-1,2-diamine (1/1) | |
Source | EPA DSSTox | |
URL | https://comptox.epa.gov/dashboard/DTXSID90669968 | |
Description | DSSTox provides a high quality public chemistry resource for supporting improved predictive toxicology. | |
Molecular Weight |
224.21 g/mol | |
Source | PubChem | |
URL | https://pubchem.ncbi.nlm.nih.gov | |
Description | Data deposited in or computed by PubChem | |
CAS No. |
172152-22-6 | |
Record name | 1,2-Propanediamine, (S)-, [S-(R*,R*)]-2,3-dihydroxybutanedioate (1:1) | |
Source | CAS Common Chemistry | |
URL | https://commonchemistry.cas.org/detail?cas_rn=172152-22-6 | |
Description | CAS Common Chemistry is an open community resource for accessing chemical information. Nearly 500,000 chemical substances from CAS REGISTRY cover areas of community interest, including common and frequently regulated chemicals, and those relevant to high school and undergraduate chemistry classes. This chemical information, curated by our expert scientists, is provided in alignment with our mission as a division of the American Chemical Society. | |
Explanation | The data from CAS Common Chemistry is provided under a CC-BY-NC 4.0 license, unless otherwise stated. | |
Record name | (2S,3S)-2,3-Dihydroxybutanedioic acid--(2S)-propane-1,2-diamine (1/1) | |
Source | EPA DSSTox | |
URL | https://comptox.epa.gov/dashboard/DTXSID90669968 | |
Description | DSSTox provides a high quality public chemistry resource for supporting improved predictive toxicology. | |
Disclaimer and Information on In-Vitro Research Products
Please be aware that all articles and product information presented on BenchChem are intended solely for informational purposes. The products available for purchase on BenchChem are specifically designed for in-vitro studies, which are conducted outside of living organisms. In-vitro studies, derived from the Latin term "in glass," involve experiments performed in controlled laboratory settings using cells or tissues. It is important to note that these products are not categorized as medicines or drugs, and they have not received approval from the FDA for the prevention, treatment, or cure of any medical condition, ailment, or disease. We must emphasize that any form of bodily introduction of these products into humans or animals is strictly prohibited by law. It is essential to adhere to these guidelines to ensure compliance with legal and ethical standards in research and experimentation.