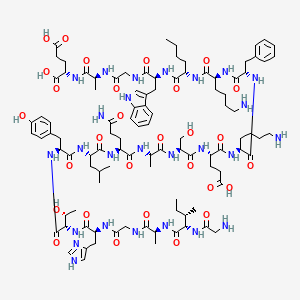
Herpes simplex virus-I proteinase substrate II
Overview
Description
Herpes simplex virus-I proteinase substrate II is a compound used in the study of herpes simplex virus type 1 (HSV-1). HSV-1 is a double-stranded DNA virus that causes infections in humans, leading to conditions such as cold sores, genital herpes, and in severe cases, encephalitis. The proteinase substrate II is specifically designed to interact with the viral protease, an enzyme critical for the virus’s replication and maturation processes.
Preparation Methods
Synthetic Routes and Reaction Conditions
The synthesis of herpes simplex virus-I proteinase substrate II involves multiple steps, including the preparation of peptide sequences that mimic the natural substrates of the viral protease. The synthetic route typically involves solid-phase peptide synthesis (SPPS), where amino acids are sequentially added to a growing peptide chain anchored to a solid resin. The reaction conditions include the use of coupling reagents like HBTU (O-Benzotriazole-N,N,N’,N’-tetramethyl-uronium-hexafluoro-phosphate) and bases like DIPEA (N,N-Diisopropylethylamine) to facilitate peptide bond formation.
Industrial Production Methods
Industrial production of this compound follows similar synthetic routes but on a larger scale. Automated peptide synthesizers are employed to increase efficiency and yield. The purification of the final product is achieved through high-performance liquid chromatography (HPLC), ensuring the compound’s purity and consistency for research applications.
Chemical Reactions Analysis
Types of Reactions
Herpes simplex virus-I proteinase substrate II primarily undergoes hydrolysis reactions catalyzed by the viral protease. The protease cleaves the peptide bonds within the substrate, leading to the formation of smaller peptide fragments.
Common Reagents and Conditions
The hydrolysis reactions typically occur under physiological conditions, with the presence of the viral protease being the key factor. The reactions are carried out in buffered solutions, often at a pH that mimics the intracellular environment of the host cells.
Major Products Formed
The major products formed from the hydrolysis of this compound are smaller peptide fragments. These fragments can be analyzed to determine the efficiency and specificity of the viral protease, providing insights into its activity and potential inhibitors.
Scientific Research Applications
Herpes simplex virus-I proteinase substrate II has several scientific research applications:
Chemistry: It is used to study the enzymatic activity of the herpes simplex virus protease, aiding in the design of inhibitors that can block viral replication.
Biology: The substrate helps in understanding the molecular mechanisms of HSV-1 infection and replication, contributing to the development of antiviral therapies.
Medicine: By identifying potential inhibitors of the viral protease, researchers can develop new treatments for HSV-1 infections, reducing the disease burden.
Industry: The compound is used in the pharmaceutical industry for high-throughput screening of antiviral drugs, accelerating the drug discovery process.
Mechanism of Action
Herpes simplex virus-I proteinase substrate II exerts its effects by mimicking the natural substrates of the viral protease. The protease recognizes and binds to the substrate, catalyzing its hydrolysis. This interaction allows researchers to study the enzyme’s activity and identify compounds that can inhibit its function. The molecular targets involved include the active site of the viral protease and the peptide bonds within the substrate.
Comparison with Similar Compounds
Similar Compounds
Herpes simplex virus-I proteinase substrate I: Another substrate used to study the viral protease, differing in its peptide sequence.
Herpes simplex virus-II proteinase substrate: Used for studying the protease of herpes simplex virus type 2 (HSV-2), which has a similar but distinct protease compared to HSV-1.
Uniqueness
Herpes simplex virus-I proteinase substrate II is unique due to its specific peptide sequence, which is optimized for interaction with the HSV-1 protease. This specificity allows for more accurate studies of the enzyme’s activity and the identification of potent inhibitors. The substrate’s design also enables high-throughput screening, making it a valuable tool in antiviral drug discovery.
Properties
IUPAC Name |
(2S)-2-[[(2S)-2-[[2-[[(2S)-2-[[(2S)-2-[[(2S)-6-amino-2-[[(2S)-2-[[(2S)-6-amino-2-[[(2S)-2-[[(2S)-2-[[(2S)-2-[[(2S)-5-amino-2-[[(2S)-2-[[(2S)-2-[[(2S,3R)-2-[[(2S)-2-[[2-[[(2S)-2-[[(2S,3S)-2-[(2-aminoacetyl)amino]-3-methylpentanoyl]amino]propanoyl]amino]acetyl]amino]-3-(1H-imidazol-4-yl)propanoyl]amino]-3-hydroxybutanoyl]amino]-3-(4-hydroxyphenyl)propanoyl]amino]-4-methylpentanoyl]amino]-5-oxopentanoyl]amino]propanoyl]amino]-3-hydroxypropanoyl]amino]-4-carboxybutanoyl]amino]hexanoyl]amino]-3-phenylpropanoyl]amino]hexanoyl]amino]hexanoyl]amino]-3-(1H-indol-3-yl)propanoyl]amino]acetyl]amino]propanoyl]amino]pentanedioic acid | |
---|---|---|
Source | PubChem | |
URL | https://pubchem.ncbi.nlm.nih.gov | |
Description | Data deposited in or computed by PubChem | |
InChI |
InChI=1S/C102H152N26O29/c1-10-12-25-66(92(146)124-75(44-61-47-108-65-26-17-16-24-64(61)65)89(143)110-49-80(134)112-56(7)87(141)121-71(102(156)157)35-38-83(138)139)116-91(145)67(27-18-20-39-103)118-96(150)73(42-59-22-14-13-15-23-59)123-93(147)68(28-19-21-40-104)117-94(148)70(34-37-82(136)137)120-99(153)77(51-129)126-88(142)57(8)113-90(144)69(33-36-78(106)132)119-95(149)72(41-53(3)4)122-97(151)74(43-60-29-31-63(131)32-30-60)125-101(155)85(58(9)130)128-98(152)76(45-62-48-107-52-111-62)115-81(135)50-109-86(140)55(6)114-100(154)84(54(5)11-2)127-79(133)46-105/h13-17,22-24,26,29-32,47-48,52-58,66-77,84-85,108,129-131H,10-12,18-21,25,27-28,33-46,49-51,103-105H2,1-9H3,(H2,106,132)(H,107,111)(H,109,140)(H,110,143)(H,112,134)(H,113,144)(H,114,154)(H,115,135)(H,116,145)(H,117,148)(H,118,150)(H,119,149)(H,120,153)(H,121,141)(H,122,151)(H,123,147)(H,124,146)(H,125,155)(H,126,142)(H,127,133)(H,128,152)(H,136,137)(H,138,139)(H,156,157)/t54-,55-,56-,57-,58+,66-,67-,68-,69-,70-,71-,72-,73-,74-,75-,76-,77-,84-,85-/m0/s1 | |
Source | PubChem | |
URL | https://pubchem.ncbi.nlm.nih.gov | |
Description | Data deposited in or computed by PubChem | |
InChI Key |
RHCBDLHUKITYRJ-HMVDRYGNSA-N | |
Source | PubChem | |
URL | https://pubchem.ncbi.nlm.nih.gov | |
Description | Data deposited in or computed by PubChem | |
Canonical SMILES |
CCCCC(C(=O)NC(CC1=CNC2=CC=CC=C21)C(=O)NCC(=O)NC(C)C(=O)NC(CCC(=O)O)C(=O)O)NC(=O)C(CCCCN)NC(=O)C(CC3=CC=CC=C3)NC(=O)C(CCCCN)NC(=O)C(CCC(=O)O)NC(=O)C(CO)NC(=O)C(C)NC(=O)C(CCC(=O)N)NC(=O)C(CC(C)C)NC(=O)C(CC4=CC=C(C=C4)O)NC(=O)C(C(C)O)NC(=O)C(CC5=CNC=N5)NC(=O)CNC(=O)C(C)NC(=O)C(C(C)CC)NC(=O)CN | |
Source | PubChem | |
URL | https://pubchem.ncbi.nlm.nih.gov | |
Description | Data deposited in or computed by PubChem | |
Isomeric SMILES |
CCCC[C@@H](C(=O)N[C@@H](CC1=CNC2=CC=CC=C21)C(=O)NCC(=O)N[C@@H](C)C(=O)N[C@@H](CCC(=O)O)C(=O)O)NC(=O)[C@H](CCCCN)NC(=O)[C@H](CC3=CC=CC=C3)NC(=O)[C@H](CCCCN)NC(=O)[C@H](CCC(=O)O)NC(=O)[C@H](CO)NC(=O)[C@H](C)NC(=O)[C@H](CCC(=O)N)NC(=O)[C@H](CC(C)C)NC(=O)[C@H](CC4=CC=C(C=C4)O)NC(=O)[C@H]([C@@H](C)O)NC(=O)[C@H](CC5=CNC=N5)NC(=O)CNC(=O)[C@H](C)NC(=O)[C@H]([C@@H](C)CC)NC(=O)CN | |
Source | PubChem | |
URL | https://pubchem.ncbi.nlm.nih.gov | |
Description | Data deposited in or computed by PubChem | |
Molecular Formula |
C102H152N26O29 | |
Source | PubChem | |
URL | https://pubchem.ncbi.nlm.nih.gov | |
Description | Data deposited in or computed by PubChem | |
DSSTOX Substance ID |
DTXSID70745552 | |
Record name | Glycyl-L-isoleucyl-L-alanylglycyl-L-histidyl-L-threonyl-L-tyrosyl-L-leucyl-L-glutaminyl-L-alanyl-L-seryl-L-alpha-glutamyl-L-lysyl-L-phenylalanyl-L-lysyl-L-norleucyl-L-tryptophylglycyl-L-alanyl-L-glutamic acid | |
Source | EPA DSSTox | |
URL | https://comptox.epa.gov/dashboard/DTXSID70745552 | |
Description | DSSTox provides a high quality public chemistry resource for supporting improved predictive toxicology. | |
Molecular Weight |
2206.5 g/mol | |
Source | PubChem | |
URL | https://pubchem.ncbi.nlm.nih.gov | |
Description | Data deposited in or computed by PubChem | |
CAS No. |
165174-61-8 | |
Record name | Glycyl-L-isoleucyl-L-alanylglycyl-L-histidyl-L-threonyl-L-tyrosyl-L-leucyl-L-glutaminyl-L-alanyl-L-seryl-L-alpha-glutamyl-L-lysyl-L-phenylalanyl-L-lysyl-L-norleucyl-L-tryptophylglycyl-L-alanyl-L-glutamic acid | |
Source | EPA DSSTox | |
URL | https://comptox.epa.gov/dashboard/DTXSID70745552 | |
Description | DSSTox provides a high quality public chemistry resource for supporting improved predictive toxicology. | |
Disclaimer and Information on In-Vitro Research Products
Please be aware that all articles and product information presented on BenchChem are intended solely for informational purposes. The products available for purchase on BenchChem are specifically designed for in-vitro studies, which are conducted outside of living organisms. In-vitro studies, derived from the Latin term "in glass," involve experiments performed in controlled laboratory settings using cells or tissues. It is important to note that these products are not categorized as medicines or drugs, and they have not received approval from the FDA for the prevention, treatment, or cure of any medical condition, ailment, or disease. We must emphasize that any form of bodily introduction of these products into humans or animals is strictly prohibited by law. It is essential to adhere to these guidelines to ensure compliance with legal and ethical standards in research and experimentation.