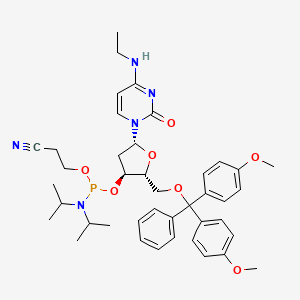
N4-Ethyl-DC cep
Overview
Description
Mechanism of Action
Target of Action
The primary target of N4-Ethyl-deoxycytidine (N4-Et-dC) is the DNA sequence, specifically the guanine (dG) base . It is used to modify oligonucleotide probes to equalize the thermal stability of guanine-cytosine (G:C) and adenine-thymine (A:T) base pairs formed upon hybridization to the target .
Mode of Action
N4-Et-dC interacts with its target by forming base pairs with dG. The n4-et-dc : dg base pair has a thermal stability similar to an a:t base pair instead of a g:c base pair .
Biochemical Pathways
N4-Et-dC affects the biochemical pathway of DNA hybridization. It is typically used to minimize the deleterious effect of large variations in guanine-cytosine (GC) content in target/probe sequences on the results produced by techniques involving simultaneous hybridization of many sequences .
Pharmacokinetics
Its impact on bioavailability is evident in its ability to normalize the thermal stability of base pairs, thereby improving the accuracy of techniques involving dna hybridization .
Result of Action
The result of N4-Et-dC’s action is the normalization of thermal stability across different base pairs. This leads to more accurate results in techniques involving simultaneous hybridization of many sequences, such as DNA chip or reverse hybridization protocols . It also helps to better understand how cytosine-guanine-containing oligos stimulate the innate immune system .
Action Environment
The action of N4-Et-dC is influenced by the environmental conditions of the DNA sequence it interacts with. For instance, sequences with high GC content may contain mismatches yet still stably hybridize due to the higher thermal stability of G:C base pairs . Conversely, low-GC content sequences may perfectly match to probe or target but the strands may dissociate upon washing . The use of N4-Et-dC helps to overcome these challenges by equalizing the thermal stability of different base pairs .
Biochemical Analysis
Biochemical Properties
N4-Ethyl-DC cep interacts with natural deoxyguanosine (dG) in DNA sequences . The N4-ethyl analogue of deoxycytidine (dC) in this compound forms base pairs with dG, but the stability of this base pair is similar to an adenine-thymine (A-T) base pair instead of a cytosine-guanine (C-G) base pair . This property is utilized to minimize the deleterious effect of large variations in GC content in target/probe sequences on the results produced by techniques involving simultaneous hybridization of many sequences .
Cellular Effects
It is known that the compound’s ability to form base pairs with dG can influence cellular processes that involve DNA hybridization .
Molecular Mechanism
The molecular mechanism of this compound involves its interaction with DNA sequences. The N4-ethyl analogue of dC in this compound forms base pairs with dG, but the stability of this base pair is reduced to about the level of an A-T base pair . This property allows this compound to influence the stability of DNA duplexes and can be used to control the results of DNA hybridization processes .
Preparation Methods
Synthetic Routes and Reaction Conditions: The synthesis of N4-Ethyl-DC cep involves the protection of the 5’-hydroxyl group of 2’-deoxycytidine with a dimethoxytrityl group, followed by the introduction of an ethyl group at the N4 position. The final step involves the attachment of a cyanoethyl phosphoramidite group at the 3’ position. The reaction conditions typically include the use of anhydrous acetonitrile as a solvent and 1H-tetrazole as an activator .
Industrial Production Methods: Industrial production of this compound follows similar synthetic routes but on a larger scale. The process involves stringent quality control measures to ensure the purity and consistency of the product. The compound is typically stored under refrigerated conditions to maintain its stability .
Chemical Reactions Analysis
Types of Reactions: N4-Ethyl-DC cep primarily undergoes substitution reactions. The ethyl group at the N4 position can be replaced under specific conditions, and the phosphoramidite group can participate in coupling reactions during oligonucleotide synthesis .
Common Reagents and Conditions:
Substitution Reactions: Common reagents include alkyl halides and strong bases.
Coupling Reactions: 1H-tetrazole or diisopropylammonium tetrazolide are commonly used as activators.
Major Products: The major products formed from these reactions are modified oligonucleotides, which incorporate this compound into their sequences .
Scientific Research Applications
N4-Ethyl-DC cep has a wide range of applications in scientific research:
Comparison with Similar Compounds
5-Methyl-2’-deoxycytidine: Another modified nucleoside used to increase the stability of DNA duplexes.
2’-Deoxyinosine: Used to introduce degeneracy in oligonucleotide sequences.
8-Oxo-2’-deoxyguanosine: Mimics oxidized guanine and is used in mutagenesis studies.
Uniqueness: N4-Ethyl-DC cep is unique in its ability to reduce the stability of the cytosine-guanine base pair to that of an adenine-thymine pair, making it particularly useful in applications requiring uniform hybridization conditions .
Properties
IUPAC Name |
3-[[(2R,3S,5R)-2-[[bis(4-methoxyphenyl)-phenylmethoxy]methyl]-5-[4-(ethylamino)-2-oxopyrimidin-1-yl]oxolan-3-yl]oxy-[di(propan-2-yl)amino]phosphanyl]oxypropanenitrile | |
---|---|---|
Source | PubChem | |
URL | https://pubchem.ncbi.nlm.nih.gov | |
Description | Data deposited in or computed by PubChem | |
InChI |
InChI=1S/C41H52N5O7P/c1-8-43-38-23-25-45(40(47)44-38)39-27-36(53-54(51-26-12-24-42)46(29(2)3)30(4)5)37(52-39)28-50-41(31-13-10-9-11-14-31,32-15-19-34(48-6)20-16-32)33-17-21-35(49-7)22-18-33/h9-11,13-23,25,29-30,36-37,39H,8,12,26-28H2,1-7H3,(H,43,44,47)/t36-,37+,39+,54?/m0/s1 | |
Source | PubChem | |
URL | https://pubchem.ncbi.nlm.nih.gov | |
Description | Data deposited in or computed by PubChem | |
InChI Key |
LGNGWXZNSIMWIS-LLVNGMGKSA-N | |
Source | PubChem | |
URL | https://pubchem.ncbi.nlm.nih.gov | |
Description | Data deposited in or computed by PubChem | |
Canonical SMILES |
CCNC1=NC(=O)N(C=C1)C2CC(C(O2)COC(C3=CC=CC=C3)(C4=CC=C(C=C4)OC)C5=CC=C(C=C5)OC)OP(N(C(C)C)C(C)C)OCCC#N | |
Source | PubChem | |
URL | https://pubchem.ncbi.nlm.nih.gov | |
Description | Data deposited in or computed by PubChem | |
Isomeric SMILES |
CCNC1=NC(=O)N(C=C1)[C@H]2C[C@@H]([C@H](O2)COC(C3=CC=CC=C3)(C4=CC=C(C=C4)OC)C5=CC=C(C=C5)OC)OP(N(C(C)C)C(C)C)OCCC#N | |
Source | PubChem | |
URL | https://pubchem.ncbi.nlm.nih.gov | |
Description | Data deposited in or computed by PubChem | |
Molecular Formula |
C41H52N5O7P | |
Source | PubChem | |
URL | https://pubchem.ncbi.nlm.nih.gov | |
Description | Data deposited in or computed by PubChem | |
Molecular Weight |
757.9 g/mol | |
Source | PubChem | |
URL | https://pubchem.ncbi.nlm.nih.gov | |
Description | Data deposited in or computed by PubChem | |
Retrosynthesis Analysis
AI-Powered Synthesis Planning: Our tool employs the Template_relevance Pistachio, Template_relevance Bkms_metabolic, Template_relevance Pistachio_ringbreaker, Template_relevance Reaxys, Template_relevance Reaxys_biocatalysis model, leveraging a vast database of chemical reactions to predict feasible synthetic routes.
One-Step Synthesis Focus: Specifically designed for one-step synthesis, it provides concise and direct routes for your target compounds, streamlining the synthesis process.
Accurate Predictions: Utilizing the extensive PISTACHIO, BKMS_METABOLIC, PISTACHIO_RINGBREAKER, REAXYS, REAXYS_BIOCATALYSIS database, our tool offers high-accuracy predictions, reflecting the latest in chemical research and data.
Strategy Settings
Precursor scoring | Relevance Heuristic |
---|---|
Min. plausibility | 0.01 |
Model | Template_relevance |
Template Set | Pistachio/Bkms_metabolic/Pistachio_ringbreaker/Reaxys/Reaxys_biocatalysis |
Top-N result to add to graph | 6 |
Feasible Synthetic Routes
Disclaimer and Information on In-Vitro Research Products
Please be aware that all articles and product information presented on BenchChem are intended solely for informational purposes. The products available for purchase on BenchChem are specifically designed for in-vitro studies, which are conducted outside of living organisms. In-vitro studies, derived from the Latin term "in glass," involve experiments performed in controlled laboratory settings using cells or tissues. It is important to note that these products are not categorized as medicines or drugs, and they have not received approval from the FDA for the prevention, treatment, or cure of any medical condition, ailment, or disease. We must emphasize that any form of bodily introduction of these products into humans or animals is strictly prohibited by law. It is essential to adhere to these guidelines to ensure compliance with legal and ethical standards in research and experimentation.