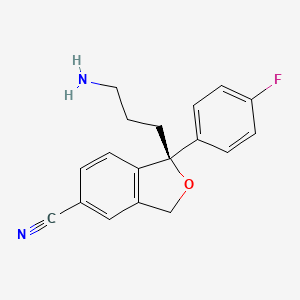
Didesmethylescitalopram
Overview
Description
Didesmethylescitalopram is an active metabolite of the antidepressant escitalopram, which is the S-enantiomer of citalopram. Like its parent compounds, this compound functions as a selective serotonin reuptake inhibitor (SSRI), contributing to the therapeutic effects of escitalopram by increasing serotonin levels in the brain .
Mechanism of Action
Target of Action
Didesmethylescitalopram is an active metabolite of the antidepressant escitalopram . Like escitalopram, it functions as a Selective Serotonin Reuptake Inhibitor (SSRI) . The primary target of this compound is the serotonin transporter (SERT) , which is responsible for the reuptake of serotonin (5-HT) in the synaptic cleft. By inhibiting this transporter, this compound increases the availability of serotonin, a neurotransmitter that plays a crucial role in mood regulation .
Mode of Action
This compound, as an SSRI, works by binding to the SERT, thereby inhibiting the reuptake of serotonin . This inhibition leads to an increase in the concentration of serotonin in the synaptic cleft, enhancing serotonergic neurotransmission . This increased serotonin availability is thought to contribute to the alleviation of depressive and anxiety symptoms .
Biochemical Pathways
The action of this compound primarily affects the serotonergic pathway . By inhibiting the reuptake of serotonin, it enhances the serotonergic transmission in the central nervous system . This can lead to secondary changes in other neurotransmitter systems, potentially affecting the overall balance of neurotransmitters in the brain .
Pharmacokinetics
It is known that its elimination half-life is approximately100 hours . This long half-life suggests that the compound stays in the body for an extended period, which could contribute to its therapeutic effects .
Result of Action
The increased serotonin availability resulting from the action of this compound can lead to improvements in mood and reductions in anxiety and depressive symptoms . As an active metabolite of escitalopram, this compound is thought to contribute to the overall therapeutic benefits of its parent drug .
Action Environment
The action of this compound, like other SSRIs, can be influenced by various environmental factors. For instance, factors such as the individual’s overall health status, the presence of other medications, and genetic factors can affect the drug’s metabolism and efficacy . .
Biochemical Analysis
Biochemical Properties
Didesmethylescitalopram, like its parent compound escitalopram, functions as a selective serotonin reuptake inhibitor (SSRI) . This means it interacts with the serotonin transporter protein, inhibiting the reuptake of serotonin into the presynaptic neuron. This results in an increase in the extracellular concentration of serotonin, enhancing serotonergic neurotransmission .
Cellular Effects
As an SSRI, this compound increases the level of serotonin in the synaptic cleft, which can affect various types of cells and cellular processes. It influences cell function by impacting cell signaling pathways, particularly those involving serotonin receptors. This can lead to changes in gene expression and cellular metabolism .
Molecular Mechanism
This compound exerts its effects at the molecular level primarily through its interaction with the serotonin transporter protein. By binding to this transporter, it inhibits the reuptake of serotonin, leading to an increase in the extracellular concentration of serotonin. This enhances serotonergic neurotransmission, which can lead to changes in gene expression .
Dosage Effects in Animal Models
The effects of this compound at different dosages in animal models are not well-studied. Ssris like escitalopram have been studied extensively. Generally, the effects of these drugs are dose-dependent, with higher doses leading to greater increases in extracellular serotonin. High doses can also lead to toxic or adverse effects .
Metabolic Pathways
This compound is involved in the metabolic pathway of the parent drug, escitalopram This likely involves various enzymes and cofactors, and could potentially affect metabolic flux or metabolite levels
Transport and Distribution
As an SSRI, it is likely to be transported into cells via the serotonin transporter, and distributed throughout the body via the bloodstream .
Subcellular Localization
As an SSRI, it is likely to be found in areas of the cell where the serotonin transporter is present, such as the presynaptic neuron .
Preparation Methods
Synthetic Routes and Reaction Conditions: Didesmethylescitalopram can be synthesized through the demethylation of escitalopram. The process involves the removal of methyl groups from escitalopram, typically using reagents such as boron tribromide (BBr3) or other demethylating agents under controlled conditions .
Industrial Production Methods: Industrial production of this compound follows similar synthetic routes but on a larger scale. The process involves the use of high-performance liquid chromatography (HPLC) for the purification of the compound, ensuring high purity and yield .
Types of Reactions:
Oxidation: this compound can undergo oxidation reactions, leading to the formation of various oxidized metabolites.
Reduction: The compound can also be reduced under specific conditions, although this is less common.
Substitution: Substitution reactions can occur, particularly involving the fluorophenyl group, leading to the formation of different derivatives.
Common Reagents and Conditions:
Oxidation: Common oxidizing agents include hydrogen peroxide (H2O2) and potassium permanganate (KMnO4).
Reduction: Reducing agents such as lithium aluminum hydride (LiAlH4) can be used.
Substitution: Reagents like halogens (e.g., chlorine, bromine) are often used in substitution reactions.
Major Products:
Oxidation: Oxidized metabolites of this compound.
Reduction: Reduced forms of the compound.
Substitution: Various substituted derivatives depending on the reagents used.
Scientific Research Applications
Didesmethylescitalopram has several scientific research applications:
Chemistry: Used as a reference compound in analytical chemistry for the study of SSRI metabolites.
Biology: Investigated for its role in modulating serotonin levels and its effects on neurotransmission.
Medicine: Studied for its potential therapeutic effects in treating depression and anxiety disorders.
Industry: Utilized in the pharmaceutical industry for the development of new antidepressant formulations.
Comparison with Similar Compounds
Desmethylcitalopram: Another metabolite of citalopram, also functions as an SSRI.
Desmethylsertraline: A metabolite of sertraline, another SSRI.
Desmethylvenlafaxine: A metabolite of venlafaxine, which is a serotonin-norepinephrine reuptake inhibitor (SNRI).
Norfluoxetine: A metabolite of fluoxetine, another SSRI.
Comparison: Didesmethylescitalopram is unique in its high selectivity for the serotonin transporter, which contributes to its therapeutic efficacy and lower side effect profile. Compared to desmethylcitalopram, this compound has a longer elimination half-life, making it more suitable for sustained therapeutic effects .
Properties
IUPAC Name |
(1S)-1-(3-aminopropyl)-1-(4-fluorophenyl)-3H-2-benzofuran-5-carbonitrile | |
---|---|---|
Source | PubChem | |
URL | https://pubchem.ncbi.nlm.nih.gov | |
Description | Data deposited in or computed by PubChem | |
InChI |
InChI=1S/C18H17FN2O/c19-16-5-3-15(4-6-16)18(8-1-9-20)17-7-2-13(11-21)10-14(17)12-22-18/h2-7,10H,1,8-9,12,20H2/t18-/m0/s1 | |
Source | PubChem | |
URL | https://pubchem.ncbi.nlm.nih.gov | |
Description | Data deposited in or computed by PubChem | |
InChI Key |
RKUKMUWCRLRPEJ-SFHVURJKSA-N | |
Source | PubChem | |
URL | https://pubchem.ncbi.nlm.nih.gov | |
Description | Data deposited in or computed by PubChem | |
Canonical SMILES |
C1C2=C(C=CC(=C2)C#N)C(O1)(CCCN)C3=CC=C(C=C3)F | |
Source | PubChem | |
URL | https://pubchem.ncbi.nlm.nih.gov | |
Description | Data deposited in or computed by PubChem | |
Isomeric SMILES |
C1C2=C(C=CC(=C2)C#N)[C@](O1)(CCCN)C3=CC=C(C=C3)F | |
Source | PubChem | |
URL | https://pubchem.ncbi.nlm.nih.gov | |
Description | Data deposited in or computed by PubChem | |
Molecular Formula |
C18H17FN2O | |
Source | PubChem | |
URL | https://pubchem.ncbi.nlm.nih.gov | |
Description | Data deposited in or computed by PubChem | |
DSSTOX Substance ID |
DTXSID30168066 | |
Record name | (S)-Didemethylescitalopram | |
Source | EPA DSSTox | |
URL | https://comptox.epa.gov/dashboard/DTXSID30168066 | |
Description | DSSTox provides a high quality public chemistry resource for supporting improved predictive toxicology. | |
Molecular Weight |
296.3 g/mol | |
Source | PubChem | |
URL | https://pubchem.ncbi.nlm.nih.gov | |
Description | Data deposited in or computed by PubChem | |
CAS No. |
166037-78-1 | |
Record name | (S)-Didemethylcitalopram | |
Source | CAS Common Chemistry | |
URL | https://commonchemistry.cas.org/detail?cas_rn=166037-78-1 | |
Description | CAS Common Chemistry is an open community resource for accessing chemical information. Nearly 500,000 chemical substances from CAS REGISTRY cover areas of community interest, including common and frequently regulated chemicals, and those relevant to high school and undergraduate chemistry classes. This chemical information, curated by our expert scientists, is provided in alignment with our mission as a division of the American Chemical Society. | |
Explanation | The data from CAS Common Chemistry is provided under a CC-BY-NC 4.0 license, unless otherwise stated. | |
Record name | Didesmethylescitalopram | |
Source | ChemIDplus | |
URL | https://pubchem.ncbi.nlm.nih.gov/substance/?source=chemidplus&sourceid=0166037781 | |
Description | ChemIDplus is a free, web search system that provides access to the structure and nomenclature authority files used for the identification of chemical substances cited in National Library of Medicine (NLM) databases, including the TOXNET system. | |
Record name | (S)-Didemethylescitalopram | |
Source | EPA DSSTox | |
URL | https://comptox.epa.gov/dashboard/DTXSID30168066 | |
Description | DSSTox provides a high quality public chemistry resource for supporting improved predictive toxicology. | |
Record name | DIDESMETHYLESCITALOPRAM | |
Source | FDA Global Substance Registration System (GSRS) | |
URL | https://gsrs.ncats.nih.gov/ginas/app/beta/substances/JM43W5556T | |
Description | The FDA Global Substance Registration System (GSRS) enables the efficient and accurate exchange of information on what substances are in regulated products. Instead of relying on names, which vary across regulatory domains, countries, and regions, the GSRS knowledge base makes it possible for substances to be defined by standardized, scientific descriptions. | |
Explanation | Unless otherwise noted, the contents of the FDA website (www.fda.gov), both text and graphics, are not copyrighted. They are in the public domain and may be republished, reprinted and otherwise used freely by anyone without the need to obtain permission from FDA. Credit to the U.S. Food and Drug Administration as the source is appreciated but not required. | |
Disclaimer and Information on In-Vitro Research Products
Please be aware that all articles and product information presented on BenchChem are intended solely for informational purposes. The products available for purchase on BenchChem are specifically designed for in-vitro studies, which are conducted outside of living organisms. In-vitro studies, derived from the Latin term "in glass," involve experiments performed in controlled laboratory settings using cells or tissues. It is important to note that these products are not categorized as medicines or drugs, and they have not received approval from the FDA for the prevention, treatment, or cure of any medical condition, ailment, or disease. We must emphasize that any form of bodily introduction of these products into humans or animals is strictly prohibited by law. It is essential to adhere to these guidelines to ensure compliance with legal and ethical standards in research and experimentation.