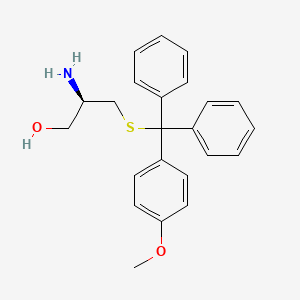
Eg5-I
Overview
Description
Preparation Methods
Synthetic Routes and Reaction Conditions
The synthesis of Eg5-I involves several steps, starting with the preparation of the core scaffold, followed by functionalization to introduce specific substituents that enhance its inhibitory activity. The synthetic route typically involves:
Formation of the core scaffold: This step involves the construction of a heterocyclic core, often through cyclization reactions.
Functionalization: Introduction of various functional groups to the core scaffold to improve binding affinity and selectivity. This may involve reactions such as alkylation, acylation, and halogenation.
Purification: The final compound is purified using techniques like column chromatography and recrystallization to obtain a high-purity product.
Industrial Production Methods
Industrial production of this compound requires optimization of the synthetic route to ensure scalability and cost-effectiveness. This involves:
Process optimization: Refining reaction conditions, such as temperature, pressure, and solvent choice, to maximize yield and minimize by-products.
Automation: Utilizing automated synthesis platforms to increase throughput and consistency.
Quality control: Implementing rigorous quality control measures to ensure the purity and potency of the final product.
Chemical Reactions Analysis
Types of Reactions
Eg5-I undergoes various chemical reactions, including:
Oxidation: this compound can be oxidized to form corresponding oxides, which may alter its biological activity.
Reduction: Reduction reactions can modify the functional groups on this compound, potentially affecting its binding affinity.
Substitution: Substitution reactions, such as nucleophilic or electrophilic substitution, can introduce new functional groups to the molecule.
Common Reagents and Conditions
Oxidation: Common oxidizing agents include hydrogen peroxide and potassium permanganate.
Reduction: Reducing agents like sodium borohydride and lithium aluminum hydride are often used.
Substitution: Reagents such as halogens, alkyl halides, and acids are employed under various conditions, including acidic or basic environments.
Major Products Formed
The major products formed from these reactions depend on the specific reagents and conditions used. For example, oxidation may yield oxides, while substitution reactions can produce a variety of substituted derivatives .
Scientific Research Applications
Eg5-I has a wide range of scientific research applications, including:
Chemistry: Used as a tool compound to study the mechanisms of mitosis and the role of kinesin spindle protein in cell division.
Biology: Employed in cellular and molecular biology research to investigate the effects of mitotic inhibition on cell cycle progression and apoptosis.
Medicine: Explored as a potential therapeutic agent for cancer treatment, particularly in cancers that exhibit high levels of kinesin spindle protein expression.
Industry: Utilized in the development of new anti-cancer drugs and as a reference compound in drug discovery and development
Mechanism of Action
Eg5-I exerts its effects by binding to the kinesin spindle protein, inhibiting its ATPase activity. This inhibition prevents the proper formation of the bipolar spindle, leading to mitotic arrest and subsequent apoptosis of the cancer cells. The molecular targets include the allosteric binding sites on the kinesin spindle protein, which are crucial for its motor activity .
Comparison with Similar Compounds
Eg5-I is compared with other kinesin spindle protein inhibitors, such as monastrol and ispinesib. While all these compounds target the same protein, this compound has unique structural features that enhance its binding affinity and selectivity. Similar compounds include:
Monastrol: The first selective inhibitor of kinesin spindle protein, known for its distinctive monoastral spindle phenotype.
Ispinesib: A potent inhibitor with high selectivity for kinesin spindle protein, used in clinical trials for cancer treatment
This compound stands out due to its improved pharmacokinetic properties and reduced off-target effects, making it a promising candidate for further development as an anti-cancer agent .
Biological Activity
Eg5, also known as kinesin spindle protein (KSP), is a motor protein that plays a crucial role in mitosis by facilitating the separation of chromosomes through the formation of a bipolar spindle. Inhibitors of Eg5, such as Eg5-I, have garnered significant attention in cancer research due to their potential as therapeutic agents. This article provides an in-depth analysis of the biological activity of this compound, including its mechanisms of action, efficacy in various cancer models, and potential clinical applications.
Eg5 functions by moving along microtubules and is essential for the proper alignment and segregation of chromosomes during cell division. Inhibition of Eg5 disrupts this process, leading to mitotic arrest and subsequent apoptosis in rapidly dividing cells. The primary mechanism through which Eg5 inhibitors operate involves the blockade of ATPase activity, which is necessary for the motor function of Eg5.
Research Findings
Recent studies have highlighted the efficacy of various Eg5 inhibitors, including YL001 and monastrol. These compounds demonstrate significant antitumor activity across multiple cancer cell lines.
Table 1: Efficacy of Eg5 Inhibitors in Various Cancer Cell Lines
Compound | Cell Line | EC50 (μM) | Observations |
---|---|---|---|
YL001 | CNE-2Z (nasopharyngeal cancer) | 5-10 | Highly sensitive to treatment |
YL001 | K562 (leukemia) | 10-15 | Significant growth inhibition |
YL001 | HT1080 (fibrosarcoma) | >70 | Resistant to treatment |
Monastrol | HeLa | 0.7 | Induces monoastral spindles |
The data indicate that the sensitivity to Eg5 inhibitors correlates with the expression levels of the Eg5 gene across different cell lines. For instance, HeLa cells exhibit higher expression levels and are more responsive to treatment compared to HT1080 cells, which show resistance due to lower expression levels of Eg5 .
Case Studies
Several case studies have illustrated the potential of Eg5 inhibitors in clinical settings:
- Multiple Myeloma : The compound Arry-520 (filanesib), an Eg5 inhibitor, has shown promising results in clinical trials for patients with multiple myeloma. It demonstrated significant efficacy in reducing tumor burden and improving patient outcomes .
- Breast Cancer : A study involving breast cancer models indicated that treatment with monastrol led to increased apoptosis and reduced tumor growth, suggesting that targeting Eg5 could be a viable strategy for therapy-resistant tumors .
- Combination Therapy : Research has indicated that combining Eg5 inhibitors with traditional chemotherapeutics may enhance overall efficacy. For example, synergy between YL001 and tubulin-targeting agents was observed, suggesting a potential strategy to overcome drug resistance .
Challenges and Future Directions
Despite the promising results associated with Eg5 inhibitors, challenges remain in their development. Many compounds exhibit limited efficacy due to the development of resistance mechanisms. Ongoing research is focused on:
- Identifying New Binding Sites : Exploring alternative binding sites on Eg5 could lead to the development of more effective inhibitors.
- Drug Synergy : Investigating combinations with existing chemotherapeutics to enhance treatment outcomes.
- Biomarker Development : Establishing biomarkers for predicting patient response to Eg5 inhibitors could facilitate personalized therapy approaches .
Q & A
Basic Research Questions
Q. What are the primary biochemical assays used to assess Eg5-I inhibitory activity, and how are their parameters optimized for reproducibility?
this compound inhibition is typically evaluated using ATPase activity assays (e.g., fluorescence-based malachite green or NADH-coupled assays) and microtubule gliding assays. Key parameters include:
- ATP concentration : Optimized between 1–5 mM to avoid substrate depletion .
- Temperature control : Maintained at 37°C to mimic physiological conditions .
- Positive controls : Use of known inhibitors (e.g., monastrol) to validate assay sensitivity . Reproducibility requires strict adherence to protocols, including pre-treatment of microtubules and normalization to vehicle controls. Raw data should include dose-response curves and IC50 calculations with error margins .
Q. Which structural features of this compound are critical for its interaction with kinesin inhibitors, and how are these characterized experimentally?
The motor domain of this compound, particularly the allosteric binding pocket near loop L5, is critical for inhibitor binding. Methods for characterization include:
- X-ray crystallography : Resolves inhibitor-bound conformations at <2.0 Å resolution .
- Site-directed mutagenesis : Identifies residues (e.g., Glu116, Tyr127) essential for binding affinity .
- Surface plasmon resonance (SPR) : Quantifies binding kinetics (kon/koff) under varying ionic conditions .
Advanced Research Questions
Q. How can researchers resolve contradictory data in this compound inhibition studies across different cellular models (e.g., cancer vs. non-cancer cell lines)?
Contradictions often arise from model-specific factors such as:
- Expression levels : Quantify this compound via Western blot or qPCR to correlate inhibition efficacy with baseline protein/mRNA levels .
- Cell cycle synchronization : Use nocodazole or thymidine block to standardize mitotic phases .
- Multi-omics integration : Combine transcriptomics (RNA-seq) and proteomics (LC-MS) to identify compensatory pathways (e.g., upregulation of KIF15) . Statistical reconciliation tools like meta-analysis or Bayesian hierarchical modeling are recommended for cross-study comparisons .
Q. What methodological approaches are recommended for analyzing this compound’s allosteric inhibition mechanisms in dynamic cellular environments?
Advanced techniques include:
- Cryo-electron microscopy (cryo-EM) : Captures conformational changes in this compound during microtubule binding (4–6 Å resolution) .
- Single-molecule fluorescence resonance energy transfer (smFRET) : Tracks real-time structural transitions under ATP hydrolysis .
- Molecular dynamics (MD) simulations : Models inhibitor-induced rigidity in the switch I/II helices over 100-ns trajectories . Data should be validated using mutant constructs (e.g., D130A) to confirm mechanistic hypotheses .
Q. How should researchers design experiments to evaluate off-target effects of this compound inhibitors in high-throughput screening (HTS) campaigns?
- Counter-screening assays : Test inhibitors against related kinesins (KIF11, KIF15) using ATPase activity assays .
- Chemoproteomics : Employ affinity-based probes (ABPs) to map inhibitor interactomes in lysates .
- Phenotypic validation : Combine siRNA knockdown and rescue experiments to isolate this compound-specific effects . A tiered approach (primary HTS → secondary specificity screens → tertiary mechanistic studies) minimizes false positives .
Q. Data Analysis and Interpretation
Q. What statistical frameworks are most robust for analyzing dose-dependent this compound inhibition in heterogeneous cell populations?
- Nonlinear regression : Fit data to a four-parameter logistic model (Hill equation) to derive IC50 and Hill slope .
- Bootstrapping : Generate 95% confidence intervals for IC50 values using 1,000+ resampled datasets .
- Cluster analysis : Segment cells by this compound expression levels (flow cytometry) to assess subpopulation-specific responses .
Q. How can researchers address variability in this compound inhibitor efficacy due to microtubule stabilization agents (e.g., paclitaxel) in combinatorial studies?
- Factorial design : Test all combinations of this compound inhibitors and stabilizers in a matrix format .
- Synergy scoring : Calculate combination indices (CI) using the Chou-Talalay method .
- Live-cell imaging : Monitor mitotic arrest duration to distinguish additive vs. antagonistic effects .
Q. Experimental Design and Validation
Q. What controls are essential for ensuring rigor in this compound knockout/knockdown experiments?
- Rescue controls : Re-express wild-type or mutant this compound in knockout models .
- Off-target controls : Include siRNA sequences with scrambled bases .
- Phenotypic benchmarks : Compare results to pharmacological inhibition (e.g., STLC treatment) .
Q. How should researchers validate computational predictions of this compound inhibitor binding modes experimentally?
- Thermal shift assays : Measure ΔTm to confirm stabilization of this compound by inhibitors .
- Isothermal titration calorimetry (ITC) : Quantify binding thermodynamics (ΔH, ΔS) .
- Cryo-EM density maps : Overlay predicted poses with experimental densities .
Q. Ethical and Reproducibility Considerations
Q. What steps are critical for ensuring ethical data reporting and reproducibility in this compound studies?
- Pre-registration : Document hypotheses and analysis plans on platforms like Open Science Framework .
- Raw data deposition : Share microscopy images, kinetic traces, and code repositories in public databases (e.g., Zenodo, GitHub) .
- MIAPE compliance : Adhere to Minimum Information About a Proteomics Experiment standards .
Properties
IUPAC Name |
(2R)-2-amino-3-[(4-methoxyphenyl)-diphenylmethyl]sulfanylpropan-1-ol | |
---|---|---|
Source | PubChem | |
URL | https://pubchem.ncbi.nlm.nih.gov | |
Description | Data deposited in or computed by PubChem | |
InChI |
InChI=1S/C23H25NO2S/c1-26-22-14-12-20(13-15-22)23(27-17-21(24)16-25,18-8-4-2-5-9-18)19-10-6-3-7-11-19/h2-15,21,25H,16-17,24H2,1H3/t21-/m1/s1 | |
Source | PubChem | |
URL | https://pubchem.ncbi.nlm.nih.gov | |
Description | Data deposited in or computed by PubChem | |
InChI Key |
WKYHDWRETJHQFE-OAQYLSRUSA-N | |
Source | PubChem | |
URL | https://pubchem.ncbi.nlm.nih.gov | |
Description | Data deposited in or computed by PubChem | |
Canonical SMILES |
COC1=CC=C(C=C1)C(C2=CC=CC=C2)(C3=CC=CC=C3)SCC(CO)N | |
Source | PubChem | |
URL | https://pubchem.ncbi.nlm.nih.gov | |
Description | Data deposited in or computed by PubChem | |
Isomeric SMILES |
COC1=CC=C(C=C1)C(C2=CC=CC=C2)(C3=CC=CC=C3)SC[C@@H](CO)N | |
Source | PubChem | |
URL | https://pubchem.ncbi.nlm.nih.gov | |
Description | Data deposited in or computed by PubChem | |
Molecular Formula |
C23H25NO2S | |
Source | PubChem | |
URL | https://pubchem.ncbi.nlm.nih.gov | |
Description | Data deposited in or computed by PubChem | |
Molecular Weight |
379.5 g/mol | |
Source | PubChem | |
URL | https://pubchem.ncbi.nlm.nih.gov | |
Description | Data deposited in or computed by PubChem | |
Disclaimer and Information on In-Vitro Research Products
Please be aware that all articles and product information presented on BenchChem are intended solely for informational purposes. The products available for purchase on BenchChem are specifically designed for in-vitro studies, which are conducted outside of living organisms. In-vitro studies, derived from the Latin term "in glass," involve experiments performed in controlled laboratory settings using cells or tissues. It is important to note that these products are not categorized as medicines or drugs, and they have not received approval from the FDA for the prevention, treatment, or cure of any medical condition, ailment, or disease. We must emphasize that any form of bodily introduction of these products into humans or animals is strictly prohibited by law. It is essential to adhere to these guidelines to ensure compliance with legal and ethical standards in research and experimentation.