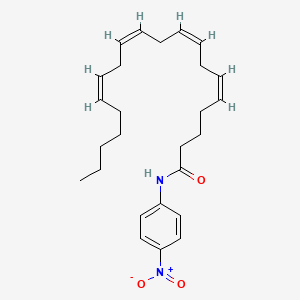
Arachidonoyl p-Nitroaniline
- Click on QUICK INQUIRY to receive a quote from our team of experts.
- With the quality product at a COMPETITIVE price, you can focus more on your research.
Overview
Description
Arachidonoyl p-Nitroaniline is a synthetic compound known for its role as a substrate in enzyme kinetic studies, particularly involving fatty acid amide hydrolase (FAAH). This compound is characterized by its long-chain unsaturated fatty acid structure, which makes it a valuable tool in biochemical research .
Mechanism of Action
Target of Action
Arachidonoyl p-Nitroaniline primarily targets the enzyme Fatty Acid Amide Hydrolase (FAAH) . FAAH is a key enzyme involved in the hydrolysis of endocannabinoids, which are bioactive lipids that regulate several neurobehavioral processes .
Mode of Action
This compound acts as a substrate for FAAH . The compound is hydrolyzed by FAAH, resulting in the release of p-nitroaniline and arachidonic acid . This interaction allows the compound to be used in enzyme kinetic studies to analyze the activity of FAAH .
Biochemical Pathways
The hydrolysis of this compound by FAAH is part of the endocannabinoid signaling pathway . Endocannabinoids like anandamide are hydrolyzed by FAAH into free arachidonic acid and ethanolamine, terminating their bioactivity . By acting as a substrate for FAAH, this compound can help elucidate the role of FAAH in this pathway .
Result of Action
The hydrolysis of this compound by FAAH results in the release of p-nitroaniline . This reaction can be measured using a spectrophotometer, providing a convenient method for assessing FAAH activity .
Biochemical Analysis
Biochemical Properties
Arachidonoyl p-Nitroaniline interacts with FAAH, an enzyme found in Dictyostelium discoideum . The compound serves as a substrate for the hydrolysis of p-nitroaniline by FAAH . This interaction is crucial for enzyme kinetic studies, such as determining the hydrolysis rate of this compound and analyzing the fatty acid amide hydrolase activity of recombinant His-FAAH purified from Dictyostelium .
Molecular Mechanism
This compound’s molecular mechanism of action is primarily through its interaction with FAAH. It serves as a substrate for the hydrolysis of p-nitroaniline by FAAH, which is a key step in certain biochemical reactions .
Metabolic Pathways
This compound is involved in the metabolic pathway related to the hydrolysis of p-nitroaniline by FAAH
Preparation Methods
Synthetic Routes and Reaction Conditions: Arachidonoyl p-Nitroaniline is synthesized through a multi-step process involving the reaction of arachidonic acid with p-nitroaniline. The synthesis typically involves the following steps:
Activation of Arachidonic Acid: Arachidonic acid is first activated using a coupling reagent such as dicyclohexylcarbodiimide (DCC) in the presence of a catalyst like 4-dimethylaminopyridine (DMAP).
Coupling Reaction: The activated arachidonic acid is then reacted with p-nitroaniline to form this compound.
Industrial Production Methods: While specific industrial production methods for this compound are not widely documented, the general approach involves scaling up the laboratory synthesis process. This includes optimizing reaction conditions, using larger reaction vessels, and ensuring stringent quality control measures to maintain product purity and consistency.
Chemical Reactions Analysis
Types of Reactions: Arachidonoyl p-Nitroaniline undergoes several types of chemical reactions, including:
Hydrolysis: Catalyzed by FAAH, leading to the release of p-nitroaniline and arachidonic acid.
Oxidation and Reduction: These reactions can modify the nitro group or the unsaturated fatty acid chain, although they are less commonly studied in this context.
Common Reagents and Conditions:
Hydrolysis: Typically involves FAAH in a buffered aqueous solution at physiological pH.
Oxidation: Can be carried out using oxidizing agents like potassium permanganate (KMnO₄) or hydrogen peroxide (H₂O₂).
Reduction: Involves reducing agents such as sodium borohydride (NaBH₄) or catalytic hydrogenation.
Major Products:
Hydrolysis: Produces p-nitroaniline and arachidonic acid.
Oxidation and Reduction: Depending on the specific conditions, products can include various oxidized or reduced derivatives of the original compound.
Scientific Research Applications
Arachidonoyl p-Nitroaniline is utilized in various scientific research fields:
Chemistry: Used to study enzyme kinetics, particularly the activity of FAAH.
Biology: Helps in understanding the metabolic pathways involving fatty acid amides.
Medicine: Investigated for its potential role in modulating endocannabinoid signaling pathways, which are implicated in pain, inflammation, and neurological disorders.
Industry: Employed in the development of biochemical assays and diagnostic tools
Comparison with Similar Compounds
Decanoyl p-Nitroaniline: Another nitroaniline fatty acid amide used to measure FAAH activity.
Oleoyl p-Nitroaniline: Similar in structure but with a different fatty acid chain, used for comparative studies in enzyme kinetics.
Uniqueness: Arachidonoyl p-Nitroaniline is unique due to its long-chain unsaturated fatty acid structure, which closely mimics natural substrates of FAAH. This makes it particularly valuable for studying the enzyme’s activity and specificity in a biologically relevant context .
Biological Activity
Arachidonoyl p-nitroaniline (ApNA) is a compound that has garnered interest in the fields of biochemistry and pharmacology due to its role as a substrate for fatty acid amide hydrolase (FAAH). This article explores its biological activity, mechanisms of action, and implications in research, particularly focusing on its anti-cancer properties and interactions with endocannabinoid systems.
Overview of this compound
This compound is classified as a nitroaniline fatty acid amide. It serves primarily as a substrate for FAAH, an enzyme responsible for the hydrolysis of various bioactive lipids, including anandamide. The hydrolysis process results in the release of arachidonic acid and p-nitroaniline, which can be quantitatively measured to assess FAAH activity .
The biological activity of ApNA is closely linked to its interaction with FAAH. When hydrolyzed by FAAH, ApNA contributes to the modulation of lipid signaling pathways. The resulting arachidonic acid can participate in the synthesis of various eicosanoids, which are crucial for inflammatory responses and cell signaling.
Hydrolysis Kinetics
The kinetics of ApNA hydrolysis can be monitored spectrophotometrically by measuring the production of p-nitroaniline at 380 nm. This method allows researchers to determine the rate of enzyme activity under various conditions, providing insights into the regulatory mechanisms governing FAAH .
Antitumor Activity
Recent studies have highlighted the potential antitumor properties associated with compounds structurally related to ApNA. For instance, DHEA (Dehydroepiandrosterone) and NALA (N-arachidonoyl-L-alanine) have been shown to induce apoptosis in head and neck squamous cell carcinoma (HNSCC) cells through mechanisms that involve increased reactive oxygen species (ROS) production and decreased phosphorylated Akt levels . This suggests that compounds like ApNA may also exhibit similar effects, potentially through receptor-independent pathways.
Table 1: Summary of Antitumor Effects in Related Studies
Compound | Cell Line | Inhibition Rate (%) | IC50 (μM) |
---|---|---|---|
DHEA | HNSCC | Variable | N/A |
NALA | HNSCC | Variable | N/A |
IMB-1406 | A549 | 100.07 | 8.99 |
IMB-1406 | HepG2 | 99.98 | 6.92 |
Note: IMB-1406 is included for comparative purposes as it showcases significant antitumor activity in similar contexts .
Role in Endocannabinoid Signaling
ApNA's role extends into the endocannabinoid system, where it may influence cannabinoid receptor signaling indirectly through its metabolic products. The inhibition of FAAH leads to increased levels of endocannabinoids, which can enhance receptor activation and downstream signaling pathways involved in pain modulation and neuroprotection .
Research Findings
- FAAH Activity Measurement : Studies have demonstrated that ApNA is effectively hydrolyzed by FAAH expressed in Dictyostelium discoideum, providing a model system for studying enzyme kinetics related to fatty acid amides .
- Reactive Oxygen Species Production : Research indicates that compounds similar to ApNA can increase ROS levels within cancer cells, leading to oxidative stress that contributes to cell death—a potential therapeutic mechanism .
- Impact on Cell Viability : In vitro assays have shown that treatments involving FAAH substrates can significantly alter cell viability in various cancer cell lines, suggesting a broader application for ApNA in cancer research .
Properties
IUPAC Name |
(5Z,8Z,11Z,14Z)-N-(4-nitrophenyl)icosa-5,8,11,14-tetraenamide |
Source
|
---|---|---|
Source | PubChem | |
URL | https://pubchem.ncbi.nlm.nih.gov | |
Description | Data deposited in or computed by PubChem | |
InChI |
InChI=1S/C26H36N2O3/c1-2-3-4-5-6-7-8-9-10-11-12-13-14-15-16-17-18-19-26(29)27-24-20-22-25(23-21-24)28(30)31/h6-7,9-10,12-13,15-16,20-23H,2-5,8,11,14,17-19H2,1H3,(H,27,29)/b7-6-,10-9-,13-12-,16-15- |
Source
|
Source | PubChem | |
URL | https://pubchem.ncbi.nlm.nih.gov | |
Description | Data deposited in or computed by PubChem | |
InChI Key |
PBMVYDNBOIVHBO-DOFZRALJSA-N |
Source
|
Source | PubChem | |
URL | https://pubchem.ncbi.nlm.nih.gov | |
Description | Data deposited in or computed by PubChem | |
Canonical SMILES |
CCCCCC=CCC=CCC=CCC=CCCCC(=O)NC1=CC=C(C=C1)[N+](=O)[O-] |
Source
|
Source | PubChem | |
URL | https://pubchem.ncbi.nlm.nih.gov | |
Description | Data deposited in or computed by PubChem | |
Isomeric SMILES |
CCCCC/C=C\C/C=C\C/C=C\C/C=C\CCCC(=O)NC1=CC=C(C=C1)[N+](=O)[O-] |
Source
|
Source | PubChem | |
URL | https://pubchem.ncbi.nlm.nih.gov | |
Description | Data deposited in or computed by PubChem | |
Molecular Formula |
C26H36N2O3 |
Source
|
Source | PubChem | |
URL | https://pubchem.ncbi.nlm.nih.gov | |
Description | Data deposited in or computed by PubChem | |
Molecular Weight |
424.6 g/mol |
Source
|
Source | PubChem | |
URL | https://pubchem.ncbi.nlm.nih.gov | |
Description | Data deposited in or computed by PubChem | |
Disclaimer and Information on In-Vitro Research Products
Please be aware that all articles and product information presented on BenchChem are intended solely for informational purposes. The products available for purchase on BenchChem are specifically designed for in-vitro studies, which are conducted outside of living organisms. In-vitro studies, derived from the Latin term "in glass," involve experiments performed in controlled laboratory settings using cells or tissues. It is important to note that these products are not categorized as medicines or drugs, and they have not received approval from the FDA for the prevention, treatment, or cure of any medical condition, ailment, or disease. We must emphasize that any form of bodily introduction of these products into humans or animals is strictly prohibited by law. It is essential to adhere to these guidelines to ensure compliance with legal and ethical standards in research and experimentation.